STE Highlights, July 2021
Awards and Recognition





- Banerjee accepted to Royal Society of Chemistry; named outstanding peer reviewer of RSC journal
- Rodgers awarded DOE NNSA Laboratory Residency Graduate Fellowship
- Kurrennoy named APS Outstanding Referee
- Winter elected member-at-large of APS GIMS
- Rao wins 2021 Innovations in Nuclear Technology R&D Award
Chemistry
New circuit-efficient quantum algorithm addresses limited qubit connectivity
Trivalent americium/neodymium work selected for Science Editor’s Choice recognition
Materials Science and Technology
Machine learning aids discovery of new double perovskite oxides
Measuring sub-surface corrosion features in plutonium storage containers
Strong interplay between defect migration and magnetic spins revealed
Awards and Recognition
Banerjee accepted to Royal Society of Chemistry; named outstanding peer reviewer of RSC journal

Amitava Banerjee
Amitava Banerjee of Materials Science in Radiation & Dynamics Extremes (MST-8) has been admitted as Royal Society of Chemistry associate member and named a 2020 top reviewer of its Journal of Materials Chemistry A.
Of the more than 51,000 active peer reviewers assessing manuscripts for the weekly journal, only 500 are recognized each year based on the number, timeliness, and quality of the reports they completed over the last year. The weekly journal focuses on novel materials related to energy and sustainability.
A Director’s Postdoctoral Fellow, Banerjee studies defect thermodynamics and kinetics pertaining to corrosion mechanisms as part of the MST-8 Radiation Science Modeling team. His work examines the complexity of the metal/oxide interface in a variety of contexts, which is important to design and tailor composite materials properties for various applications.
Banerjee earned his master of technology in materials science and engineering from the Indian Institute of Technology in Kanpur, India, and his doctoral degree in energy conversion and storage from Uppsala University in Uppsala, Sweden.
Technical contact: Amitava Banerjee
Rodgers awarded DOE NNSA Laboratory Residency Graduate Fellowship

Brian Rodgers
Colorado School of Mines graduate student Brian Rodgers is set to take advantage of the experimental science opportunities available at Los Alamos National Laboratory beginning this fall.
Rodgers received a DOE NNSA Laboratory Residency Graduate Fellowship (LRGF) and will work with Saryu Fensin, Dynamic and Quasi-Static Loading (experimental) team leader in Materials Science in Radiation and Dynamics Extremes (MST-8). Rodgers will perform molecular dynamics simulations augmented by machine learning to determine the solid-liquid interfacial energy of aluminum-silver of different crystallographic orientations with varying alloy compositions.
Experimental methods to measure the interfacial energy of a solid and a liquid of the same composition are difficult to perform and prone to error. Molecular dynamics can determine this material parameter but require time, expertise, and powerful computers, all of which are available at LANL. During his fellowship, which consists of a minimum of two 12-week periods, Rodgers will perform the simulations and design and implement experiments to validate the results.
While this information is crucial to understanding and predicting the microstructures developed during solidification in this particular alloy, this project sets the stage in development of a toolkit that can be used to predict processing-structure relationships, a key goal within the manufacturing area of leadership for LANL.
Rodgers, who earned his master’s degree in metallurgical and materials engineering in 2019, is due to receive his doctoral degree in spring 2023. His research aims to develop a deeper understanding of the solidification behavior and microstructural development during additive manufacturing.
Launched in 2017, the LRGF program connects students pursuing doctoral degrees in fields of study that address complex science and engineering problems critical to stewardship science with scientists at DOE NNSA facilities.
Technical contact: Saryu Fensin
Kurrennoy named APS Outstanding Referee
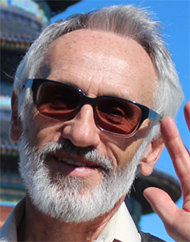
Sergey Kurennoy
Sergey Kurennoy of Accelerators and Electrodynamics (AOT-AE) has been recognized by the American Physical Society (APS) as a 2021 outstanding referee for his volunteer work maintaining the rigorous peer-review process for the Physical Review journals.
As leader of AOT-AE’s Beam Physics Modeling Team, Kurennoy researches electromagnetic problems in accelerators and beam dynamics and works on novel projects such as proton energy boosters. Before joining the Lab in 1996, he was a guest scientist at the Superconducting Super Collider Laboratory in Dallas, Texas, and a research scientist in accelerator physics at the University of Maryland. Kurennoy earned his doctoral degree in theoretical and mathematical physics from the Institute for High Energy Physics in Russia, where he was also a scientist.
Each year APS editors select around 150 outstanding referees from roughly 71,000 current referees based on the quality, number, and timeliness of their reports, without regard for APS membership, country of origin, or field of research.
Technical contact: Sergey Kurennoy
Winter elected member-at-large of APS GIMS
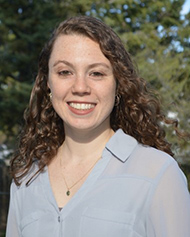
Laurel Winter
Laurel Winter of the National High Magnetic Field Laboratory-Pulsed Field Facility (MPA-MAGLAB) was elected member-at-large of the American Physical Society (APS) Topical Group on Instrument and Measurement Science (GIMS).
GIMS was formed in 1984 to provide professional recognition to those who advance instrument and measurement science in all fields of physics.
As a member of the GIMS executive committee, she will support its mission to advance the development of new instrumentation and measurement science and to provide a forum for discussion of these topics.
Her three-year-term runs through March 2024, during which she will serve primarily as a liaison between the membership and the executive committee.
Winter is the Pulsed Field Facility’s user program director. At the LANL Magnet Lab, she focuses on condensed matter physics at low temperatures and high magnetic fields with an emphasis on strongly correlated electron systems and heavy fermions. She received a bachelor’s degree in physics from Clark University in 2009 and a doctoral degree in physics from Florida State University in 2015.
Technical contact: Laurel Winter
Rao wins 2021 Innovations in Nuclear Technology R&D Award

Ashwin Rao
First Lieutenant Ashwin Rao, a guest scientist at LANL and a doctoral candidate at the Air Force Institute of Technology (AFIT), has received the 2021 Innovations in Nuclear Technology R&D Award. This award is given by the Department of Energy (DOE) to a student who makes significant R&D contributions to nuclear technology. The award is competed for across the AFIT, the Air Force Research Laboratory, and other U.S. academic institutions.
Rao’s work at LANL led to the award and is focused on machine learning techniques applied to Laser Induced Breakdown Spectroscopy (LIBS). He was hosted by the Actinide Analytical Chemistry group and mentored by scientists John Auxier II and Dung Vu.
Rao is a U.S. Air Force physicist on active duty since 2018. His research involves investigating novel analytical methods and tools to conduct chemometric analysis of nuclear materials, particularly plutonium alloys. His main focus is LIBS to acquire optical emission spectra of nuclear materials and using machine learning techniques to perform chemical analysis of the material based on spectral information. Rao previously earned a bachelor’s degree in nuclear engineering and radiological sciences from the University of Michigan in 2018 and a master’s degree in nuclear engineering from AFIT in 2020.
Technical contact: Ashwin Rao
Chemistry
New circuit-efficient quantum algorithm addresses limited qubit connectivity
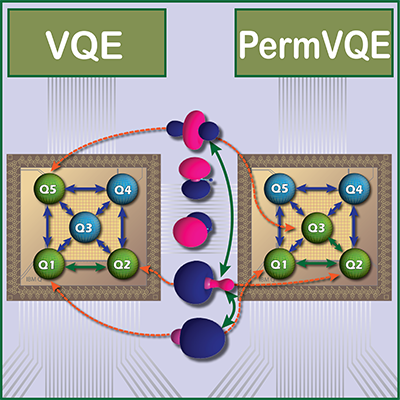
Symmetries offer simple solutions to complex problems: A permutation scheme allows solving high complexity problems in quantum chemistry with simple and short quantum circuits.
Los Alamos researchers have developed a new circuit-efficient quantum algorithm to solve electronic structure problems in quantum chemistry using near-term gate-based quantum computers. This new method is expected to facilitate the simulation of larger molecular systems devices and ultimately significantly advance studies of quantum chemical states and thus chemistry as a whole. The work of the Los Alamos team was just published in the journal PRX Quantum.
Quantum chemistry and quantum computers
One of the major goals of quantum chemistry is the calculation of the molecular electronic ground and excited state energies and corresponding wave functions of electrons from first-principles. Such eigenvalues and eigenvectors can be obtained from the solution of the time-independent electronic Schrődinger equation. However, since the invention of classical digital computers in the early 1940s, the exact numerical solution of this central quantum mechanical equation remains infeasible for systems having more than 12 electrons distributed on 184 spin-orbitals. The reason is that the solution space grows factorially with the system size (e.g., the number of electrons and basis functions). One of the most promising and immediate applications of quantum computers is solving classically intractable quantum chemistry problems.
Currently a hybrid algorithm called the variational quantum eigensolver (VQE) is the best means of modeling the ground and excited states of molecules on near-term noisy intermediate-scale (NISQ) quantum devices. Although successful implementation of VQE on quantum computers has been shown across several “toy” molecular systems, the anticipated benefits of quantum computing have yet to be fully realized.
In their recent work, the Los Alamos team presents a novel procedure for performing VQE calculations with lower depth circuits. In quantum computing, a qubit (or quantum bit) is the base unit of information, analogous to the conventional computer bit. Quantum computing architectures have limited qubit connectivity, i.e., one physical qubit is only coupled to a few neighbor qubits. Consequently, many quantum “gates” are needed to connect remote qubits. These gates introduce noise to the computer’s operation and make chemical simulations inefficient. Thus, the mapping of electronic structure problems in qubit space onto the physical qubits is critical for achieving better accuracy and computing efficiency.
The new Los Alamos algorithm, abbreviated as permutation VQE (PermVQE), allows for efficient reordering of qubits and thus overcomes some of the limitations of current quantum computing architectures. The new PermVQE algorithm finds the optimal ordering of qubits. Consequently, the algorithm is able to reduce number of gates required for performing quantum chemistry simulations, which in turn allows noise to be reduced.
Funding and mission
Research presented in this article was supported by the Laboratory Directed Research and Development (LDRD) program of LANL under Project No. 20200056DR. Additional support comes from U.S. Department of Energy (DOE), Office of Science, Office of Advanced Scientific Computing Research, under the Accelerated Research in Quantum Computing program. This work was conducted, in part, at the Center for Nonlinear Studies and the Center for Integrated Nanotechnologies (CINT), DOE, Office of Basic Energy Sciences user facility at LANL. The research supports the Laboratory’s Global Security mission area and the Integrating Information, Science, and Technology for Prediction Capability Pillar in the category “Quantum Computing” and Lab Agenda: 2.4 Assert leadership in the National Quantum Initiative.
The Los Alamos team included researchers from LANL’s Inorganic Isotope and Actinide Chemistry Group (C-IIAC), Physics and Chemistry of Materials Group (T-1), Physics of Condensed Matter and Complex Systems Group (T-4), and Accelerators and Electrodynamics Group (AOT-AE).
Reference
“Correlation-Informed Permutation of Qubits for Reducing Ansatz Depth in the Variational Quantum Eigensolver,” PRX Quantum, 2, 020337 (2021). DOI: https://doi.org/10.1103/PRXQuantum.2.020337. Authors: Nikolay V. Tkachenko (C-IIAC), James Sud (T-4), Yu Zhang (T-1), Sergei Tretiak (T-1), Petr M. Anisimov (AOT-AE), Andrew T. Arrasmith (T-4), Patrick J. Coles (T-4), Lukasz Cincio (T-4) and Pavel A. Dub (C-IIAC).
Technical contacts: Yu Zhang (T-1), Lukasz Cincio (T-4) and Pavel Dub (C-IIAC)
Trivalent americium/neodymium work selected for Science Editor’s Choice recognition
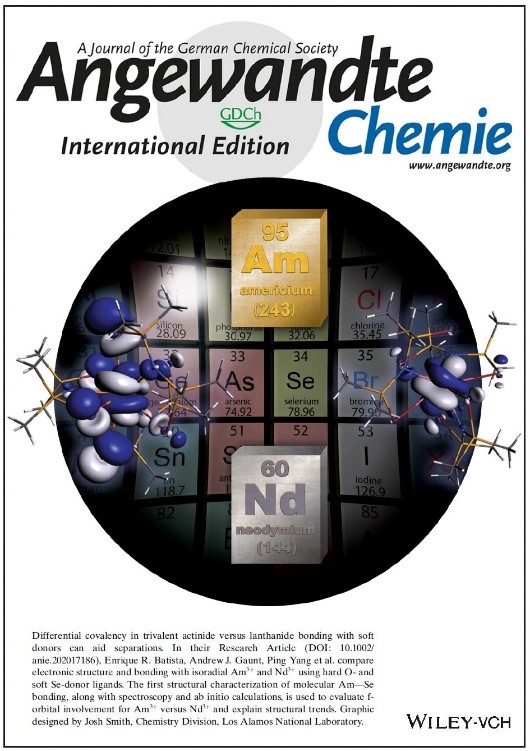
Differential covalency in trivalent actinide versus lanthanide bonding with soft donors can aid separations.
LANL work on trivalent americium/neodymium complexes was selected by Science magazine for Editor’s Choice recognition on April 8, 2021. The research was originally published in the journal Angewandte Chemie and featured on the back cover.
The actinides americium and curium are significant contributors to the long-term radiological burden of used nuclear fuel, leading to proposals for their separation and transmutation into less hazardous isotopes. However, the trivalent oxidation state dominates their chemistry and make separation based on redox chemistry difficult.
Studies by Los Alamos scientists on bonding in americium and neodymium complexes using UV-vis-NIR spectroscopy and single-crystal X-ray structures has led to a greater understanding of differences in f-orbital involvement between Am–Se and Nd–Se bonds and sheds light on this important problem. The researchers have performed the first structural characterization of molecular Am–Se bonding along with spectroscopy and ab initio calculations of f-orbital involvement for Am vs Nd and explain structural trends.
Funding and Mission
This work supports the Laboratory’s Energy Security Solutions mission and the Materials for the Future Pillar. Funding was provided by DOE Basic Energy Sciences Heavy Element Chemistry Program, the Center for Actinide Science and Technology (a DOE EFRC), a J. R. Oppenheimer Postdoctoral Fellowship, and a G.T. Seaborg Postdoctoral Fellowship.
Reference
“Structural and spectroscopic comparison of soft-Se vs hard-O donor bonding in trivalent americium/neodymium molecules,” Angewandte Chemie 60, 9459 (2021); doi.org/10.1002/anie.202017186. Authors: Goodwin, Conrad A.P., Schlimgen, Anthony W., Albrecht-Schönzart, Thomas E., Batista, Enrique R., Gaunt, Andrew J., Janicke, Michael T., Kozimor, Stosh A., Scott, Brian L., Stevens, Lauren M., White, Frankie D., and Yang, Ping.
Materials Physics and Applications
Assessing the state of non-hybrid redox flow batteries
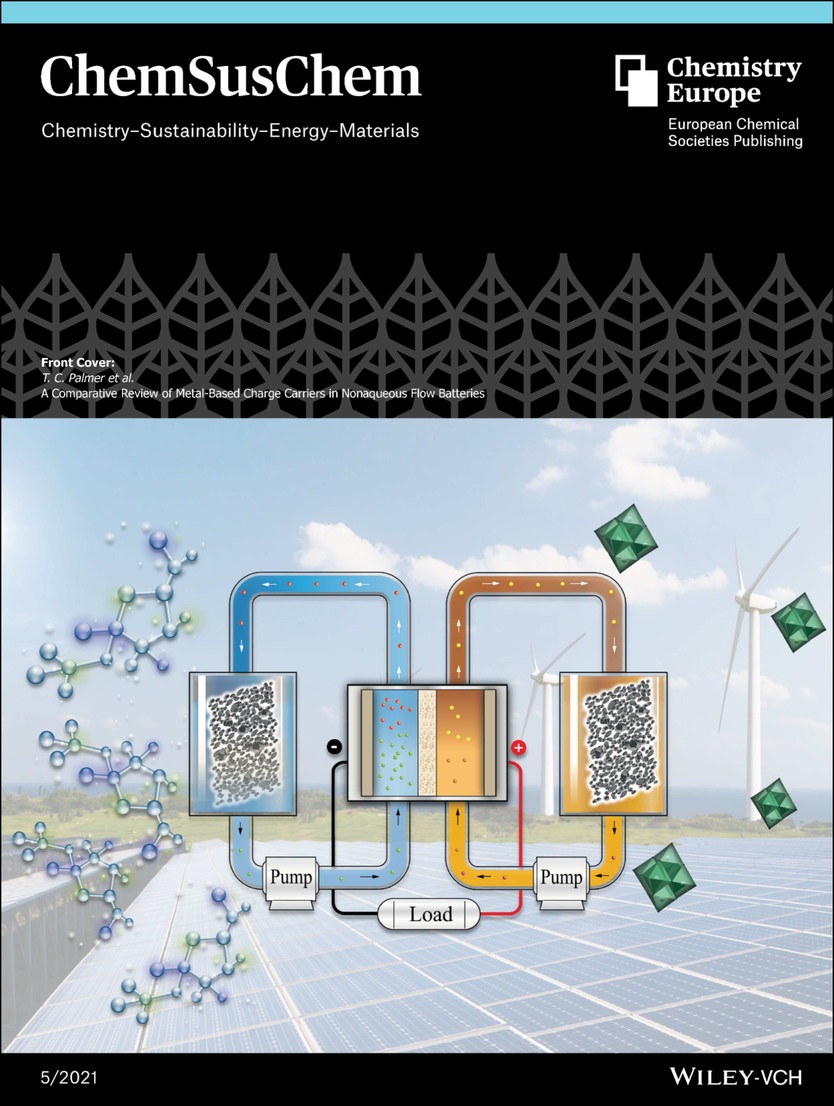
The work of Los Alamos researchers and collaborators at SNL was featured on the cover of ChemSusChem, volume 14, issue 5, in March 2021.
Given the intermittent nature of renewable energy generation, storage technology is a key requirement for grid stability and further incorporation of solar and wind sources. Nonaqueous redox flow batteries may be part of the solution, if particular key technological barriers are overcome.
In work featured on the cover of ChemSusChem, Los Alamos researchers and their collaborators at Sandia National Laboratories (SNL) presented a comprehensive review on the state of the art of metal-based nonaqueous redox flow batteries (RFB). Nonaqueous RFBs have a much larger electrochemical window, enabling higher voltage and greater energy density compared with aqueous RFBs. RFB technologies may meet the need for long-duration storage not available in other electrical energy storage devices, such as Li-ion batteries.
The team compared recent progress in experimental and theoretical computational modeling towards improving the performance of molecular, macromolecular, and redox-targeted systems. Lead authors Ben Davis and Travis Palmer of Materials Synthesis and Integrated Devices (MPA-11), Travis Anderson (SNL), and the team identified many challenges related to improving key chemical and design parameters that remain to be solved. Increasing voltage to take advantage of the entire electrochemical window of organic solvents and improving the reversibility of redox events to increase the service lifetime of RFBs are the chief hurdles for this technology. The team concluded that ultimately, if progress continues at the current rate, a viable system for grid-scale application could become reality in the near future.
The researchers highlight recent advancements from three areas within this field: 1) the evolution of high-voltage molecular charge carriers (MCCs), 2) macromolecular systems capable of storing large numbers of electrons, and 3) redox-targeted systems to circumvent solubility limitations. Despite the first examples being described in the 1980s, MCCs have only seen limited development, with most examples exhibiting poor cyclability. Macromolecular systems have been investigated heavily in the last decade and overcome the cyclability of MCCs; unfortunately, their large size has limited solubility and practical applications. Lastly, redox-targeted systems have bypassed the need for high solubility; however, this approach still faces challenges stemming from the complexities of surface area and electron transfer kinetics between solid state and dissolved moieties.
Mission and Funding
The Los Alamos portion of the work was supported by Los Alamos’s Laboratory Directed Research and Development (LDRD) Program and a J. Robert Oppenheimer Distinguished Postdoctoral Fellowship. The work supports the Lab’s Energy Security mission and its Materials for the Future science pillar.
Reference
“A comparative review of metal-based charge carriers in nonaqueous flow batteries,” ChemSusChem, 14, 1214 (2021); https://doi.org/10.1002/cssc.202002354. Authors: Travis C. Palmer, Andrew Beamer, Tristan Pitt, Benjamin L. Davis (MPA-11); Enrique R. Batista (Center for Nonlinear Studies, T-CNLS); Ping Yang, Ivan A. Popov (Physics and Chemistry of Materials, T-1),); Claudina X. Cammack, Harry D. Pratt III, Travis M. Anderson (SNL).
Technical contact: Benjamin Davis
Materials Science and Technology
Machine learning aids discovery of new double perovskite oxides
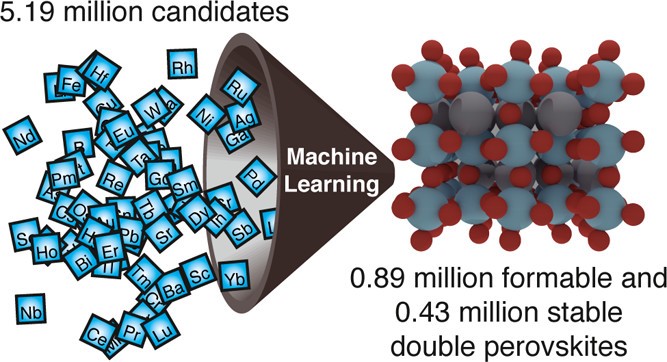
The study identifies (1) atomic features relevant to prediction of formability and stability in perovskite and double perovskite compounds, (2) the importance of including energy contributions due to local structural relaxations going beyond the high symmetry perovskite phase, and (3) 437,828 double perovskite compounds that are likely to be stable and 891,188 compounds that are likely to be formable.
Perovskite oxides continue to attract interest due to their fascinating and wide-ranging properties for diverse applications. The tunability of these properties may be further enhanced by increasing their compositional complexity via double perovskite-ordered configurations containing multiple cations.
In work featured in Chemistry of Materials, Los Alamos materials scientists applied machine learning and first principles computations in tandem to explore an exhaustive chemical space of single and double oxide perovskites. Demonstrating the utility of its machine learning approach, the team identified over 400,000 likely stable compositions with 414 that are particularly promising candidates for future experimental synthesis of novel oxide perovskites, significantly extending the chemical space of known perovskites that can be considered for a range of applications.
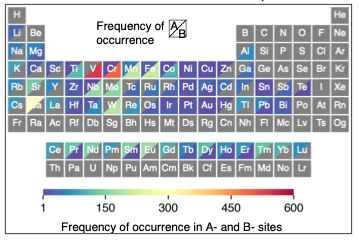
The machine learning approach identified 414 novel cubic double perovskite chemistries spanning the periodic table with high confidence.
More generally, the study highlights the potential for using machine-learning-guided high-throughput calculations to identify novel chemistries that may exhibit enhanced functionality. Thus, it serves as the first step in a more comprehensive research plan in which the functionality of promising compounds is examined, opening new avenues for materials designed for new and novel applications.
The team examined the relationship between formability, the practical ability to synthesize a compound, and stability, the thermodynamic preference to form the structure. Formability and stability training data sets were developed using existing experimental literature and in-house density functional theory computations, containing 1,505 and 3,469 examples, respectively, which represents state of the art in the current open literature in perovskite and double perovskite compounds. Subsequently, cross validated and highly accurate machine learning classification models were built using these training data sets and employed to screen for novel stable oxide perovskites.
Mission and funding
The Laboratory Directed Research and Development (LDRD) Program funded the work, which supports the Lab’s Energy Security mission and its Materials for the Future and Information Science and Technology capability pillars. In particular, the team’s machine learning approach is part of Los Alamos’s efforts to develop advanced and new materials by intentionally controlling their functionality and predicting their performance, the key goal of the Materials for the Future strategy. The Lab’s high performance computing clusters provided computational support.
Reference
“A machine learning approach for the prediction of formability and thermodynamic stability of single and double perovskite oxides,” Chemistry of Materials 33, 845 (2021). DOI: https://doi.org/10.1021/acs.chemmater.0c03402. Authors: Anjana Talapatra, Blas P. Uberuaga, Christopher R. Stanek, and Ghanshyam Pilania, Materials Science in Radiation and Dynamics Extremes (MST-8).
Technical contact: Anjana Talapatra
Measuring sub-surface corrosion features in plutonium storage containers

Figure 1. A reconstructed 3D image of three corrosion features along the inside surface of the container near the weld region. This image shows the three major features observed on the surface of one of the samples.
Researchers at Los Alamos National Laboratory (LANL), Savannah River National Laboratory (SRNL), and other Department of Energy (DOE) sites are working to better understand corrosion in stainless steel storage containers used to store excess plutonium-bearing materials. A LANL team has developed an imaging technique to assess subsurface cracks in containers for the first time and begin to predict the lifetime of the container – crucial information for ensuring the safe storage of plutonium materials.
Plutonium is packaged across the entire DOE complex in 3013 containers designed to safely store plutonium metals and oxide for up to 50 years for use in future missions. Unfortunately, corrosion pits have been observed on the inside surface of the containers storing hydrated chloride-bearing plutonium. Surface microscopy can be used to measure the pits’ depths, but provides no information about the sub-surface extent.

Figure 2. A high resolution inverted sub-surface image at Bellatrix. (The feature is rendered positive, in that the steel is transparent and the surfaces are rendered solid). This image shows the intricate corrosion ramifications within the sub-surface that cannot be seen on the surface image.
To measure the extent of sub-surface damage, a surveilled destructive examined (DE) 3013 container, previously packaged at Hanford with hydrated chloride-bearing plutonium oxide materials, was opened at Savannah River National Laboratory (SRNL) and cut into several pieces for nondestructive 3D imaging using x-ray computed tomography. With this technique, 2D reconstructed slices and 3D renderings of not only the surface corrosion pits, but also the sub-surface cracks, are possible.

Figure 3. A single slice through the corrosion at Bellatrix. This image shows extent of the corrosion with features extending into the sub-surface for ~300 µm.
The samples were imaged at LANL in the Engineered Materials Group (MST-7) using the Versa 520 micro-x-ray computed tomography instrument to visualize the depth and amount of corrosion cracking with a ~2 µm voxel size. Due to the potential contamination, the samples were kept double bagged and x-ray imaged in situ. Figure 1 is a reconstructed 3D image of three corrosion features along the inside surface of the container near the weld region. Figure 2 is a higher resolution image of one of the features. The renderings are inverted in that the steel is transparent and the surfaces and void space are rendered solid. Figure 3, a single reconstructed slice through the corrosion is shown. With these images, the researchers can measure the depth of the pits and the extent of the corrosion (~300 µm for this one in particular).
Mission and Funding
This work was funded by the Material Identification & Surveillance (MIS) program, led by LANL Program Manager Laura Worl, Pit Production Mission Integration (PPMI-DO), which supports the entire DOE complex. The team included Juan G. Duque (Physical Chemistry & Applied Spectroscopy, C-PCS), Brian M. Patterson and Lindsey Kuettner (MST-7), Daniel Rios (Materials Recovery & Recycle, AMPP-4) and other members of the MIS team. The Nuclear Materials Science Group (MST-16) provided transport and packaging support.
Technical contacts: Brian M. Patterson and Juan G. DuqueStrong interplay between defect migration and magnetic spins revealed
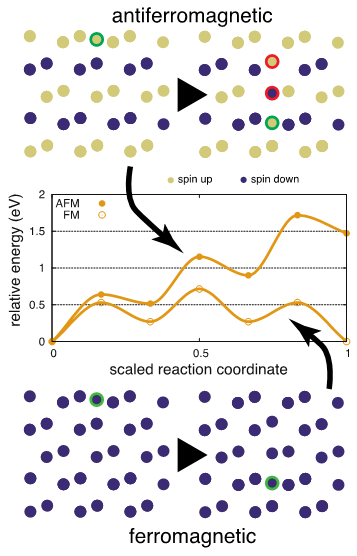
In work featured in Physical Review Materials, LANL materials scientists described the oft-unstudied coupling between defect migration and magnetic spins in oxides relevant for corrosion and energy applications. Their investigation revealed that tuning and/or controlling spins could also control mass transport, and that spin configuration can affect the migration energy rate, a key factor impacting corrosion and radiation damage effects in materials.
Magnetic materials are ubiquitous in a wide range of technological applications, from electrical generation to memory devices. However, precise techniques for creating novel magnetic devices require an understanding of mass transport and the defects that mediate it. Further, many of the same materials are present in nuclear systems, and predicting their evolution under corrosive and irradiative environments also necessitates understanding defect migration.
Critically, the LANL team, in investigating tuning and spin control, found that the magnetic spin configuration in the oxide and the migration energy of the migrating defect change in concert to modify one another. This holds profound implications for mass transport in magnetic materials. For example, understanding the evolution of rust (Fe2O3) may require knowing the magnetic spin configuration of the atoms in the material. The work also has implications for tuning magnetic random-access memory devices, where controlled defects (useful for high-density memory and neuromorphic computing applications), uncontrolled defects (useful for security applications as they can generate randomness and increase the entropy), and spin-alignment (useful for spin-transfer torque magnetic random-access memory) all are crucial factors.
The team used density functional theory calculations to determine the migration energy of cation interstitials in both magnetic and non-magnetic corundum-structured oxides. An antiferromagnetic structure in which spins are anti-aligned, and a ferromagnetic structure in which they are aligned, were both considered for Fe2O3 — an important oxide in energy materials and in the corrosion of iron-based materials. The team found that as the interstitial migrates, it can leave behind misaligned spins in the matrix, which, in turn, change the energy landscape of the interstitial. The migration energy of defects will thus be quite sensitive to the underlying magnetic structure and the rate at which misaligned spins relax. Comparing two limits in which the spins can or cannot relax, the migration energy for cation interstitial migration changed by 1 eV. At room temperature, this would mean a difference of about 1016 in migration rate, which would have an astronomical effect on the rate at which matter flows through the system, with profound consequences on corrosion and radiation damage evolution in such materials.
Mission and funding
This work was primarily supported by FUTURE (Fundamental Understanding of Transport Under Reactor Extremes), an Energy Frontier Research Center funded by the DOE, Office of Science, Basic Energy Sciences. The Laboratory Directed Research and Development program supported efforts to calculate the energetics of spin flips in the matrix. This research leveraged the Lab’s expertise and capabilities in materials modeling and thin film characterization and used resources provided by the LANL Institutional Computing program. The research supports the Lab’s Energy Security mission and its Materials for the Future science pillar — particularly the themes of Defects and Interfaces and Extreme Environments — by probing pathways to developing materials with controlled functionality and predictable performance, the key aim of the Lab’s Materials for the Future strategy.
Reference
“Interplay between defect transport and cation spin frustration in corundum-structured oxides,” Physical Review Materials, 5, 034410 (2021); DOI: https://doi.org/10.1103/PhysRevMaterials.5.034410. Authors: Amitava Banerjee, Aaron A. Kohnert, Blas P. Uberuaga (Materials Science in Radiation and Dynamics Extremes, MST-8); and Edward F. Holby (Electrochemistry and Corrosion, Sigma-2).Theoretical
A natural way to follow multiple pathways during photoexcited reactions
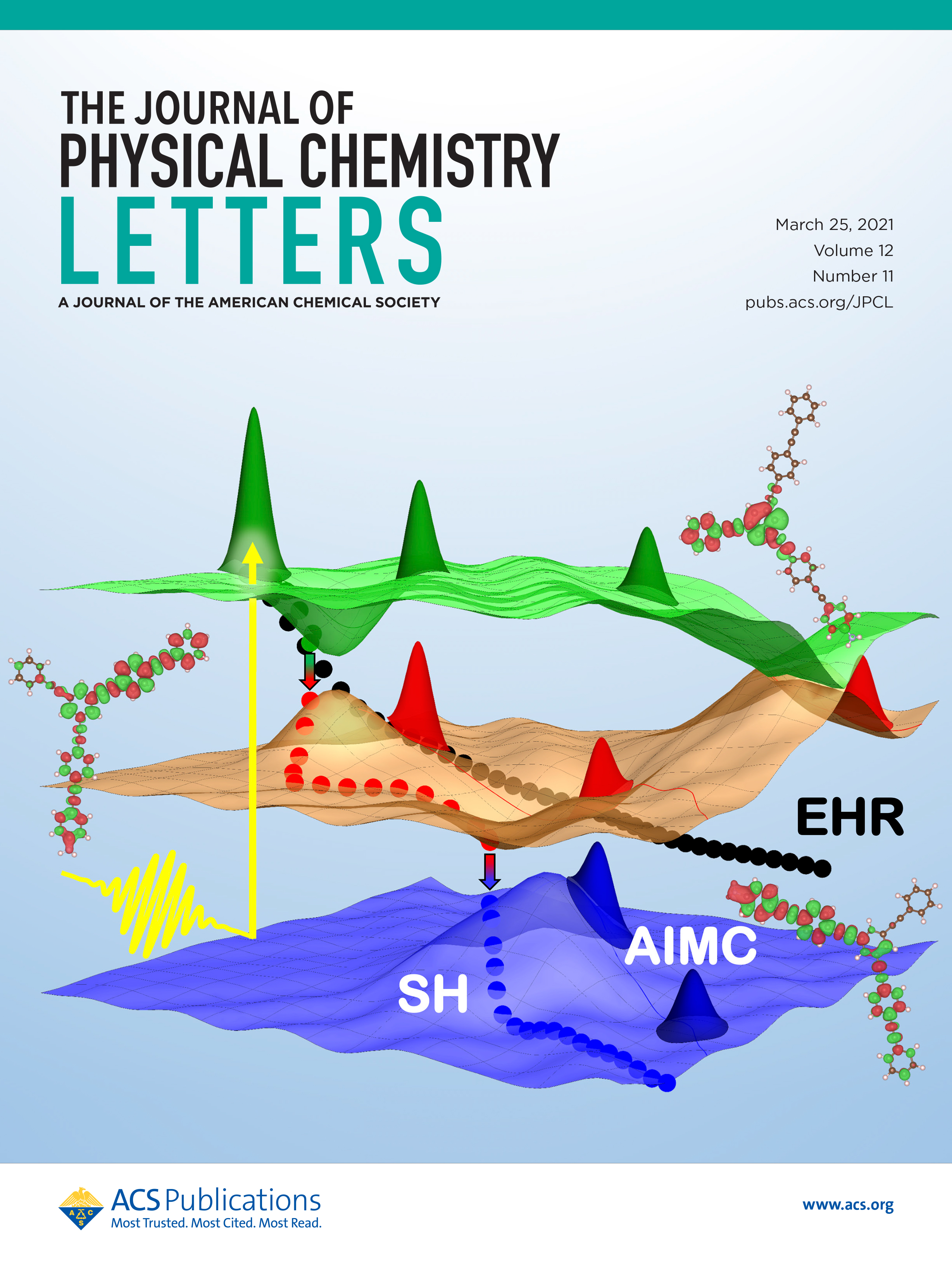
Nonadiabatic dynamics methods (AIMC, SH, and EHR) differ in the way nuclei evolve on potential energy surfaces and in the way the quantum (electronic) and classical (nuclear) systems are coupled. While SH considers a single surface with discrete quantum transitions, AIMC incorporates the branching of wave packets to the mean-field EHR.
The researchers at the Center for Integrated Nanotechnology (CINT) and the Theoretical Division have collaborated with the University of Leeds and Universidad Nacional de Quilmes, Argentina to develop and implemented an advanced excited state nonadiabatic molecular dynamics (NAMD) algorithm building on the well-known Ehrenfest mean field (EHR) approach.
EHR assumes that nuclei move classically following a force that is an average of all electronic states. The approach suffers from an inability to follow branching reaction pathways where more than one product can be formed, but cannot be described by a single mean force field. To overcome this inability, the new NAMD approach combines two developments. First, the multiconfigurational Ehrenfest (MCE) approach uses many individual EHR trajectories to guide the center of Gaussian basis functions. Second, the ab initio multiple cloning (AIMC) sampling technique improves the Gaussian basis. Each EHR trajectory is allowed to clone into two copies when crossing states have sufficiently different PESs. Each time the trajectory is cloned, the original EHR trajectory is replaced with two new trajectories and does not change the wave function. Both configurations then evolve independently.
The new multiconfigurational algorithm is implemented in LANL’s NEXMD (Nonadiabatic EXcited state Molecular Dynamics) package and DOE’s primary quantum chemistry software NWChem (maintained by Pacific Northwest National Laboratory) to provide the quantitative and accurate simulation methods for theoretically modeling nonadiabatic dynamics processes.
The traditional methods to treat nonadiabatic dynamics included the Surface-Hopping (SH), which allows the nuclei to stochastically jump between different electronic potential energy surfaces (PESs) by a computed transition probability, or the Ehrenfest (EHR) algorithm to calculate the Mean-Field average forces from multiple PESs. Both methods can provide some physical essence of the nonadiabatic process but disregard (SH) or overestimate (EHR) the interaction between different potential energy surfaces and only consider a single pathway in the simulation. Therefore, the introduction of an advanced ab initio multiple cloning (AIMC) algorithm breaks this bottleneck.
By means of all three nonadiabatic dynamics methods (AIMC, SH, and EHR) and their implementation at different levels of sophistication (NEXMD and NWChem), the atomistic and electronic structure information can now be obtained from the excited state molecular dynamics simulations of a broad variety of chemical systems and photoexcited reactions ranging from semi-classical approaches to multiconfiguration ab initio dynamics.
Funding and mission
The research at LANL was funded by Laboratory Directed Research and Development (LDRD) and the U.S. DOE, Office of Science, Basic Energy Sciences, Chemical Sciences, Geosciences, and Biosciences Division. This research was supported by the Center for Nonlinear Studies (CNLS), the Center for Integrated Nanotechnologies (CINT), and the LANL Institutional Computing (IC) Program. The research supports the Lab’s Materials for the Future and Information Science and Technology capability pillars.
References
“Nonadiabatic Excited-State Molecular Dynamics Methodologies: Comparison and Convergence,” Journal of Physical Chemistry Letters, 12, 2970-2982 (2021): doi: 10.1021/acs.jpclett.1c00266. Authors: Victor M. Freixas, Alexander J. White, Tammie Nelson, Huajing Song, Dmitry V. Makhov, Dmitrii Shalashilin, Sebastian Fernandez-Alberti, and Sergei Tretiak.
“An Ab Initio Multiple Cloning Method for Non-Adiabatic Excited-State Molecular Dynamics in NWChem,” Journal of Chemical Theory and Computation, 17, 3629–3643 (2021); doi: 10.1021/acs.jctc.1c00131. Authors: Huajing Song, Victor M. Freixas, Sebastian Fernandez-Alberti, Alexander J. White, Yu Zhang, Shaul Mukamel, Niranjan Govind, and Sergei Tretiak
Technical contacts: Tammie Nelson, Alex White, Huajing (Wilson) Song, Sergei Tretiak