STE Highlights, January 2021
Awards and Recognition
Four LANL scientists rank in 2020 list of ‘Highly Cited Researchers'
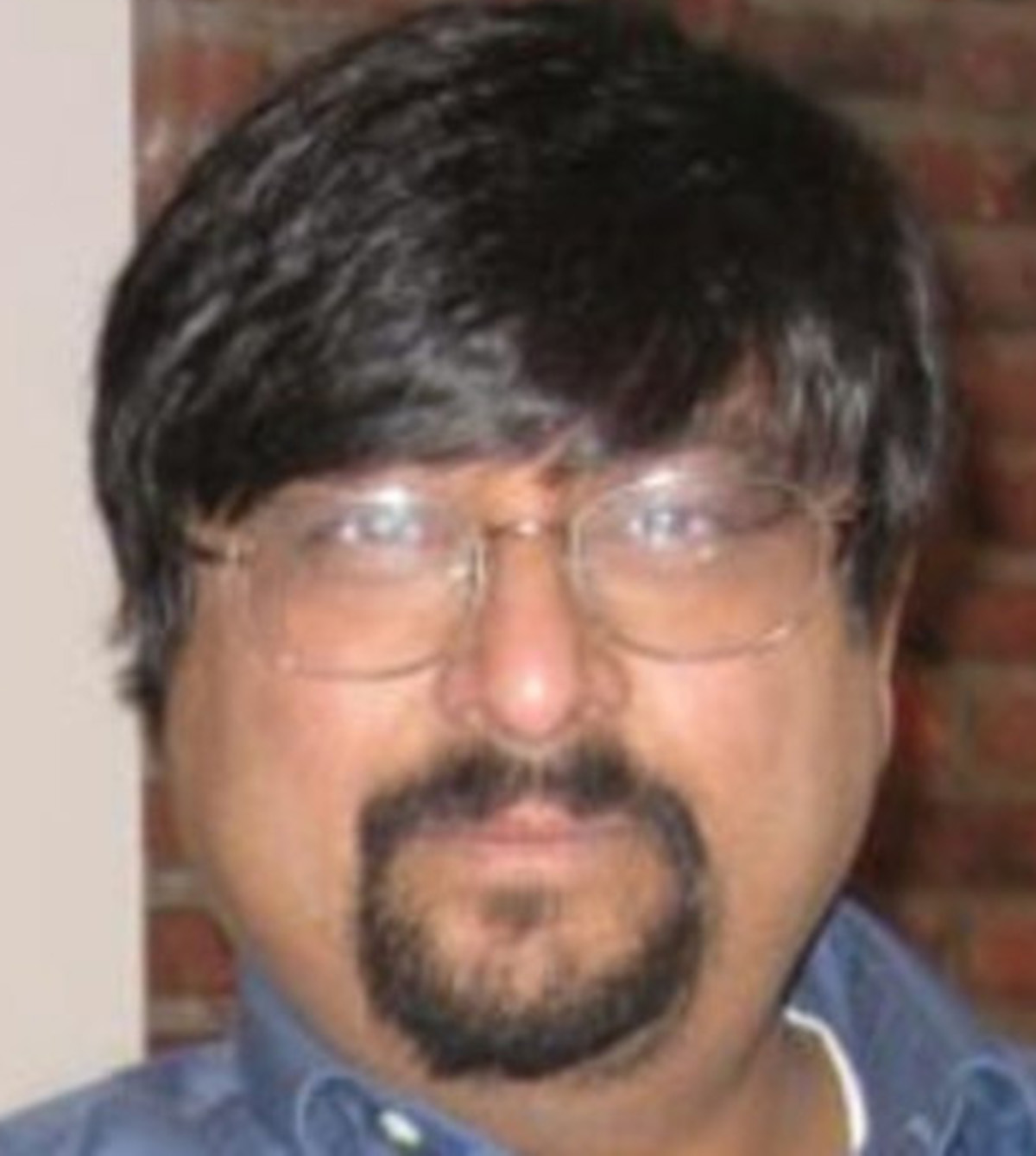
Tanmoy Bhattacharya
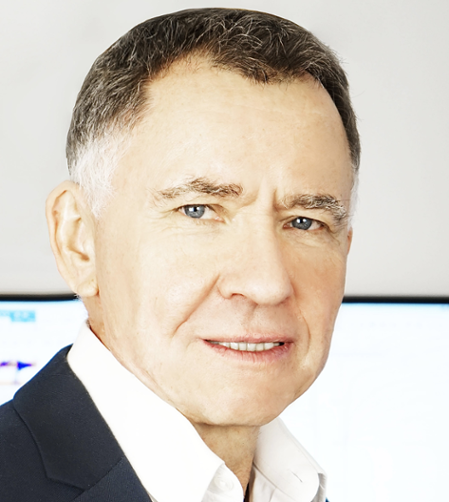
Victor I. Klimov
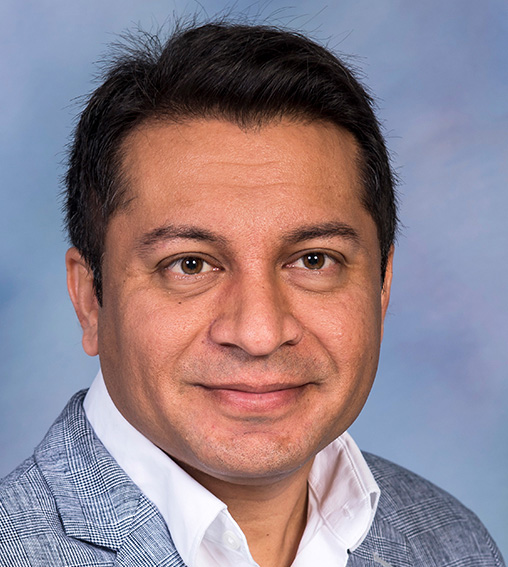
Aditya Mohite
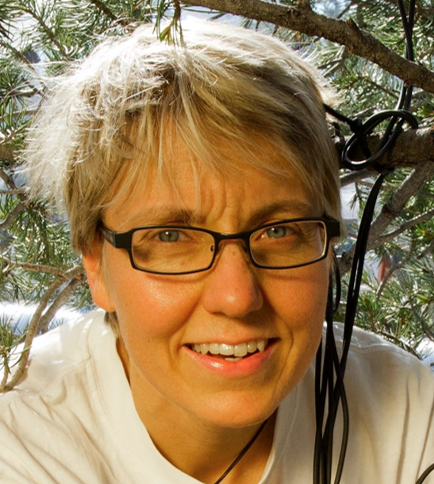
Sanna Sevanto
The Web of Science offers a niche platform for searching scientific content, where annually the search data is collated to determine the top-cited researchers of the year. In 2020, four researchers from Los Alamos National Laboratory ranked among the leaders.
Tanmoy Bhattacharya (Nuclear & Particle Physics/Astrophysics & Cosmology, T-2), Victor I. Klimov (Physical Chemistry & Applied Spectroscopy, C-PCS), Aditya Mohite (formerly Materials Physics and Applications, currently Rice University), and Sanna Sevanto (Earth System Observations, EES-14) each published papers that ranked in the top 1% of citations by their peers. These highly cited papers demonstrate the quality and scientific influence of the researcher as well as the reach of the work.
Bhattacharya, Klimov, and Mohite were previously ranked in the 2019 Highly Cited Researchers list—all categorized as cross-field influence. Sevanto was added to the list in 2020 under the Plant and Animal Science category, carried by her Plant, Cell & Environment paper, “How trees die? A test of the hydraulic failure and carbon starvation hypotheses.”
Much of Sevanto’s work has been published in specialist journals, but her core research, which is devoted to understanding vegetation responses to drought, continues to be brought front and center to the public’s attention through recent extreme drought events and severe forest fires.
“I was surprised and delighted to receive the honor,” says Sevanto. “The surprise stems from having mostly published my work in specialist journals rather than journals like Nature, Science, or PNAS. It is great to see that people find the important information they need to forward their work from these specialist journals.”
Bhattacharya’s top paper is his contribution to the Proceedings of the National Academy of Sciences: “Identification and characterization of transmitted and early founder virus envelopes in primary HIV-1 infection.”
Klimov’s most-cited papers document the discoveries of colloidal quantum dot lasing (Science, “Optical gain and stimulation emission in nanocrystal quantum dots,” 2,299 citations), carrier multiplication (Physical Review Letters, “High efficiency carrier multiplication in PbSe nanocrystals: Implications for solar energy conversion,” 1,467 citations), and quantized Auger recombination (Science, “Quantization of multiparticle Auer rates in semiconductor quantum dots,” 1,048 citations).
Mohite’s top paper was also published in Science: “High-efficiency solution-processed perovskite solar cells with millimeter-scale grains.”
Since 2001, the Highly Cited Researchers list has identified global research scientists demonstrating exceptional influence. Los Alamos continues to rank among the top.
Capability Enhancement
Unsupervised AI software is like a data science Swiss Army knife
Data science struggles with the balance between acquiring larger, higher-dimensional data sets and ensuring that data is still useful. For many, big data can mean big problems because there aren’t enough sophisticated and robust tools to aid in extracting features, which are often key to scientific progress.
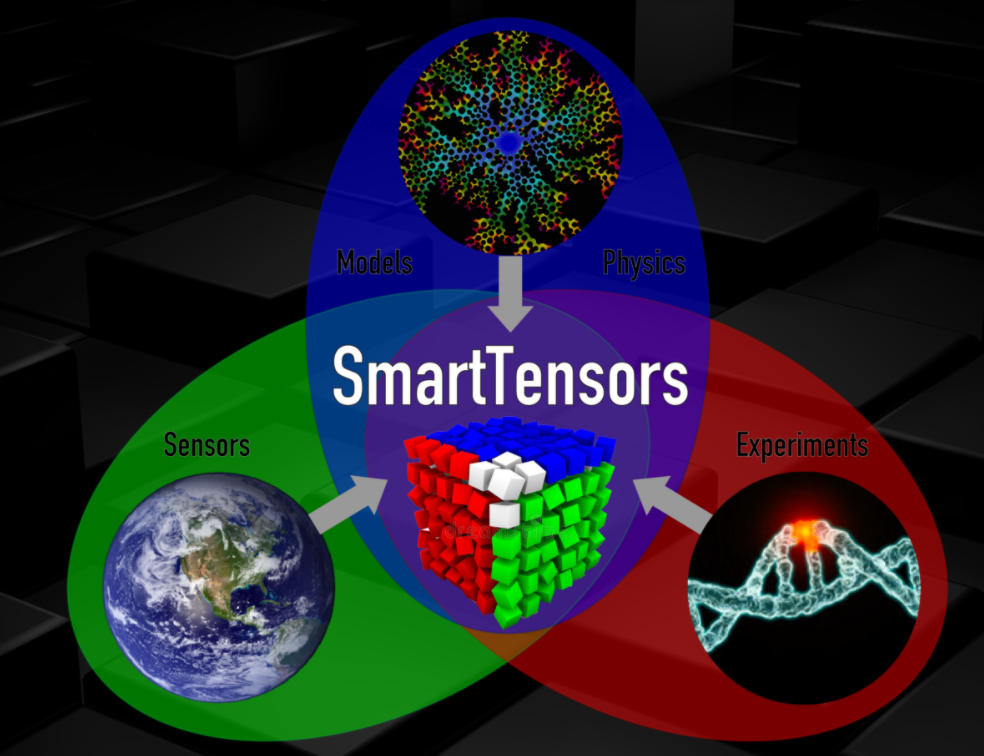
An overview diagram of the next-generation SmartTensors tool.
To mitigate this issue, Los Alamos researchers developed a novel software tool that employs unsupervised artificial intelligence to sift through massive data sets and discover latent trends, mechanisms, signatures, and features hidden in the data.
“With our tool, we can extract latent patterns in cancer genomics, protein structure, chemical reactions, and micro-climates. But we can also find hidden features for fraud detection, macro-economics, compressed images, and text mining,” says Boian Alexandrov of Theoretical Division (T-1).
This software is equivalent to a Swiss Army knife—a tool that provides a solution for almost any problem, as long as that problem pertains to big data.
“Unsupervised artificial intelligence allows for unbiased analyses that aren’t impacted by data labeling, subject-matter-expert opinions, or physics assumptions,” says Velimir (Monty) Vesselinov of Earth and Environmental Sciences (EES-16).
Watch a video: Novel Machine Learning Methods for Extraction of Features Characterizing Complex Datasets and Models
Alexandrov and Vesselinov co-created this novel, robust approach that harnesses the power of mathematical tensor factorization to extract data trends invisible to the traditional supervised artificial intelligence methods. Their unsupervised tensor method is far more powerful for feature extraction because it does not rely on training and examples—significant limitations for supervised methods.
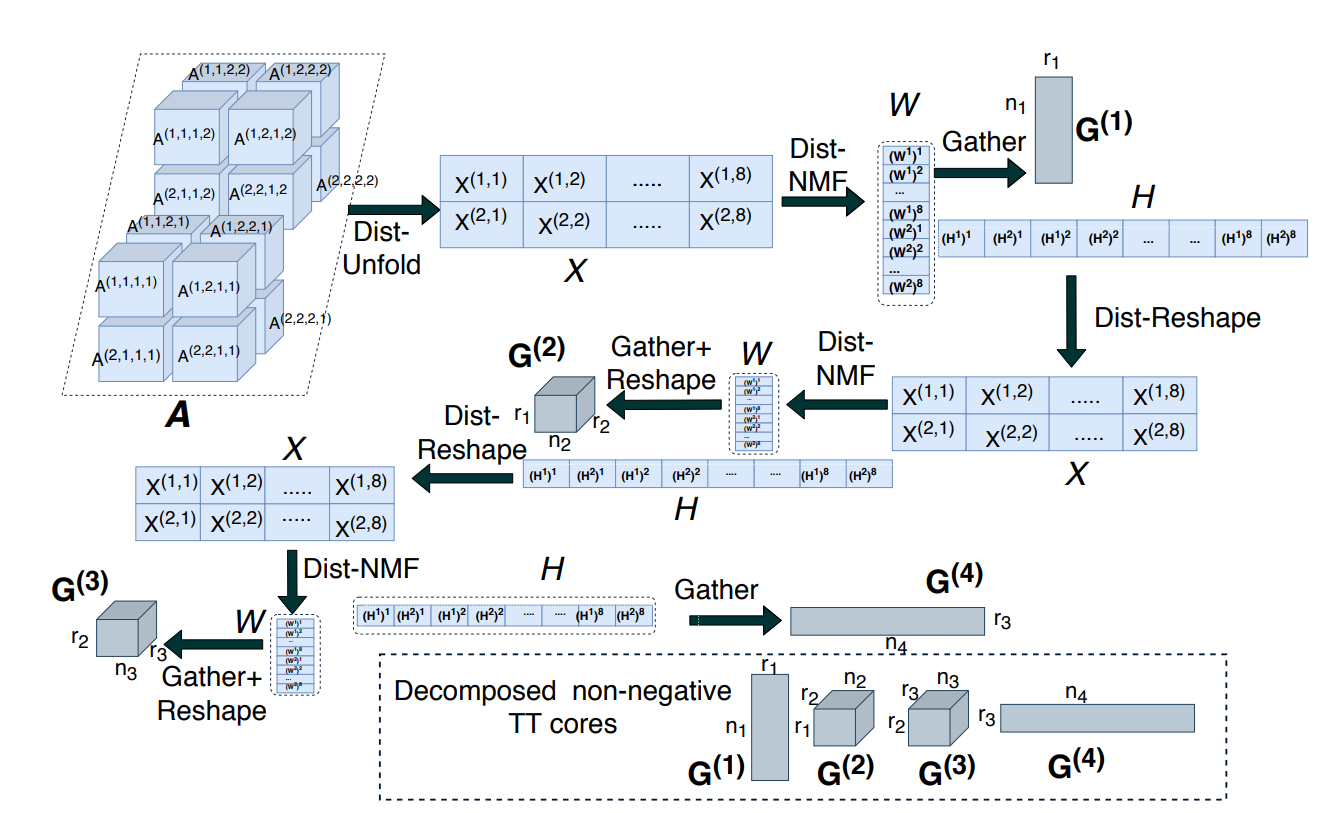
An overview diagram of the next-generation tensor-train decomposition of a 4D tensor with a processor grid of size 2 x 2 x 2 x 2.
The novel unsupervised method also unearths unknown dependencies, such as between model inputs and outputs. Additionally, this tool can go beyond discovering latent features hidden in the data: this unrivaled tool can also make predictions based on the extracted hidden features and the gained knowledge of how they are intertwined in the data.
Since the inception of the unsupervised methodology, Alexandrov and Vesselinov have designed next-generation open-source tools that accelerate computations and make the methodology more widely accessible. These new tools have been widely used, nationally and internationally, illustrating the predictive power of the methodology as well as its data compression and image de-noising capabilities. As data becomes more complex, both in terms of data set size and data dimensionality, the need for tools that can efficiently compress that data down to a reasonable size and extract meaningful information from it becomes more relevant each day.
The novel tool can be applied to emerging fields, such as personalized medicine, as well as key areas of interest for national security, such as nuclear, space, and climate science. Additionally, the tool can run on both classical supercomputers and quantum annealers—a rather unique feat.
Funding and mission
This research was funded by a LANL Laboratory Directed Research and Development (LDRD) award. The work supports the Laboratory’s Nuclear Deterrence mission area and the Integrating Information, Science, and Technology for Prediction capability pillar.
References:
Chennupati, R. Vangara, E. Skau, H. Djidjev, and B. S. Alexandrov. “Distributed non-negative matrix factorization with determination of the number of latent features.” J. Supercomputing (2020). https://doi.org/10.1007/s11227-020-03181-6Alexandrov, B., M. Karimi, V. Petkova, J. M. Asara, M. Griffin, and A. Usheva. “Aberrant cardiac energy metabolism in metabolic syndrome: insight from metabolomics and a pig model.” Scientific Reports (2020). doi: 10.1038/s41598-020-60387-7
Further resources: https://github.com/SmartTensors and http://tensors.lanl.gov
Technical contacts: Boian Alexandrov and Velimir (Monty) Vesselinov
Earth and Environmental Sciences
Novel tech leads to highest-resolution material characterization and internal defect ultrasonic imaging
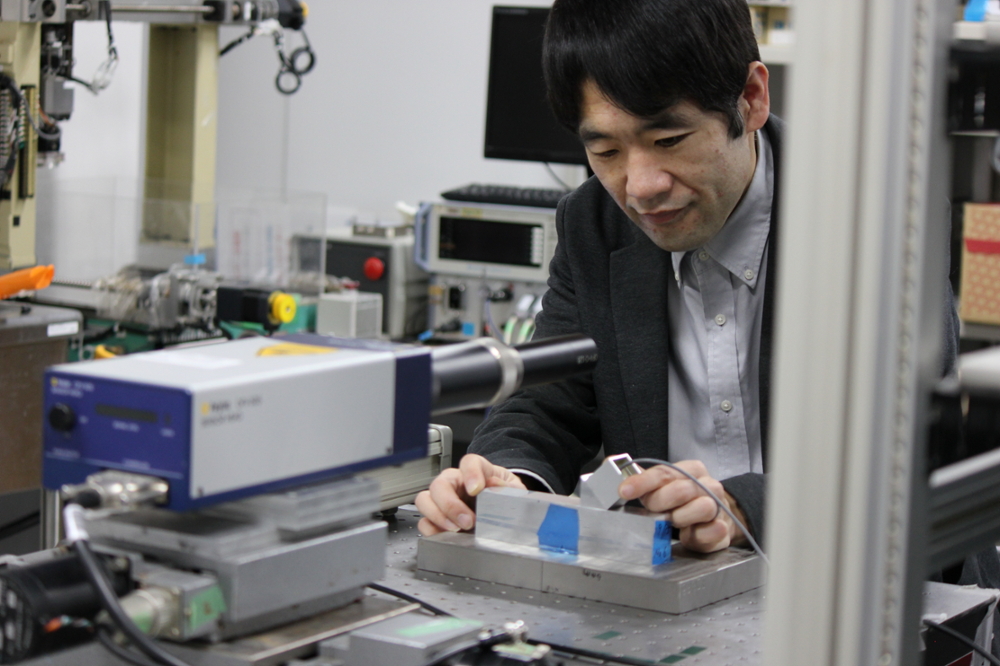
Crack-wave dynamics are very complex, so the more information you can capture about the waves, the more detailed the imaging will be. Here, Yoshikazu Ohara of Tohoku University, a Los Alamos collaborator, sets up the PLUS experiment in front of a laser.
A new 3D ultrasound imaging system combines non-contact lasers, broadband detection, and phased array technology for the first time. The result is the highest-resolution ultrasound images ever obtained of internal material cracks and defects.
The proof of concept for the novel technology was demonstrated via simulation, and more recently, the Los Alamos and collaborating Tohoku University developers published experimental results in the journal Applied Physics Letters.
“We built upon the established technique of phased array by adding a piezoelectric and laser ultrasonic system, which we call PLUS,” says Marcel Remillieux of Environmental and Earth Sciences (Geophysics, EES-17).
With PLUS, the researchers demonstrate the high-resolution imaging power—on the order of thousands of elements, which is impossible to achieve with a conventional piezoelectric matrix array transducer. PLUS provides a higher signal-to-noise ratio and can be implemented over a wider range of frequencies than traditional methods.
“This technology fills the previous information gap and can be applied to numerous industries: oil and gas, nuclear energy, and aerospace, for example,” says T.J. Ulrich of Detonation Science and Technology (Q-6).
Being able to peek inside industrial components to see if there are defects without damaging or destroying the component in any way is extremely advantageous. It boosts the quality control without increasing costs or sacrificing inventory. This is of particular benefit to the Los Alamos national security mission.
The researchers are pursuing industry collaborations through the Feynman Center for Innovation to take the technology to the next level. A patent has already been filed.
Funding and mission
This research was funded by the Japan Society for the Promotion of Science KAKENHI and the Used Fuel Disposition and Nuclear Energy University Partnerships programs. The work supports the Laboratory’s Nuclear Deterrence mission area and the Complex Natural and Engineered Systems capability pillar.
References: Yoshikazu Ohara, Marcel C. Remillieux, Tomomi Onuma, Kosuke Tsunoda, Toshihiro Tsuji, and Tsuyoshi Mihara. “Toward an ultra-high resolution phased-array system for 3D ultrasonic imaging of solids.” Appl. Phys. Lett. 117, 111902 (2020); doi: 10.1063/5.0021282
“PLUS Takes 3D Ultrasound Image of Solids,” Tohoku University News
Technical contact: Marcel Remillieux
Two studies improve understanding movement of methane
Two new studies out of the LANL Earth and Environmental Sciences Division (EES) investigate the movement of methane. The first study focuses on harvesting this natural resource for use, such as heating homes. The second study approaches methane movement from a different perspective, highlighting how free movement (without being captured) can impact environmental systems.
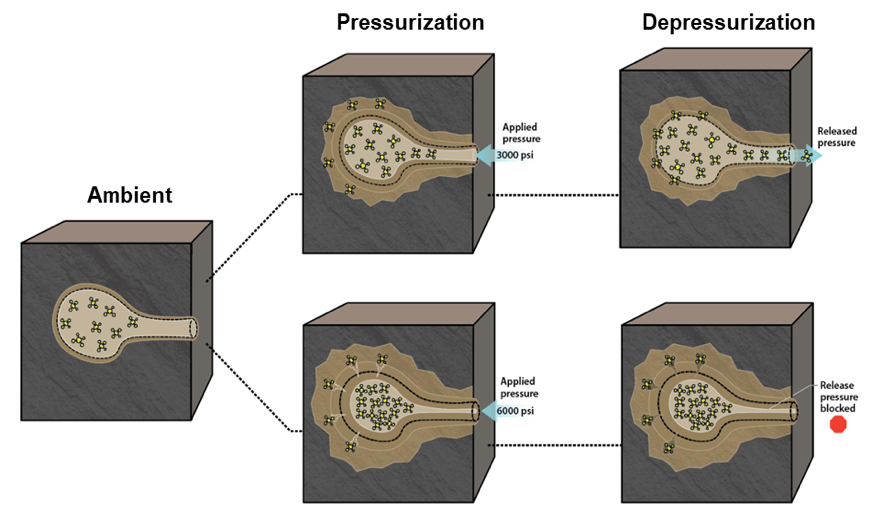
Proposed mechanism for dense methane trapping in nanopores within a kerogen matrix. At higher pressures (6,000 psi), irreversible deformation of the kerogen matrix results in methane retention in pores, even after depressurization.
First study: Improving recovery of natural gas
In this study, Los Alamos researchers reveal a new strategy to more easily access methane, a valuable energy resource. The study contradicts conventional wisdom about how methane (natural gas) is trapped in rock.
Most of the U.S. natural gas is hidden deep within shale reservoirs. Low shale porosity and permeability make recovering natural gas in tight reservoirs challenging. The pores are miniscule—typically less than five nanometers—and poorly understood. Maximizing recovery thus necessitates a better understanding of hydrocarbon interactions in the shale matrix, where fluid flow can be significantly different due to nano-confinement.
The EES team integrated molecular dynamics simulations with novel in situ high-pressure small-angle neutron scattering (SANS) conducted at the NIST Center for Neutron Research (NCNR) to examine gas transport and recovery as pressure is modified to extract methane from Marcellus shale (originating from the nation’s largest natural gas field).
The investigation focused on interactions between methane and kerogen organic content in rock, which stores a majority of hydrocarbons. The team discovered that while high pressures are beneficial for methane recovery from larger pores, dense gas is trapped in smaller, abundant shale nanopores due to kerogen deformation. For the first time, the researchers present experimental evidence that this deformation exists, and the team proposed a methane-releasing pressure range that can significantly impact methane recovery at hydraulic fracturing field sites.
Methane behavior was compared during two pressure cycles with peak pressures of 3,000 psi and 6,000 psi, as it was previously believed that increasing pressure from injected fluids into fractures would increase gas recovery.
Combining experimental observations with previously modeled kerogen mechanical behavior at high pressures, the team discovered that unexpected methane behavior occurs in very small but prevalent nanopores in the kerogen; the pore uptake of methane was elastic and reversible during drawdown from the 3,000 psi pressure peak but became plastic and irreversible at 6,000 psi, causing permanent kerogen deformation and irreversibly trapping methane clusters in the sub-2 nanometer kerogen pore space (90 percent of the measured shale porosity). These insights enrich our understanding of confined fluids behavior and help optimize the operational parameters that maximize hydrocarbon recovery.
Funding and mission
This work was supported by the Department of Energy, Office of Fossil Energy, the National Energy Technology Laboratory, and the LANL Laboratory Directed Research and Development (LDRD) Program. The work supports the Laboratory’s Energy Security mission area and the Complex Natural and Engineered Systems capability pillars.
References
Neil, C.W., Mehana, M., Hjelm, R.P., Hawley, M.E., Watkins, E.B., Mao, Y., Viswanathan, H., Kang, Q., and Xu, H., 2020a. Reduced methane recovery at high pressure due to methane trapping in shale nanopores. Nature’s Communications Earth & Environment, https://doi.org/10.1038/s43247-020-00047-w
Neil, C.W., Hjelm, R.P., Hawley, M.E., Watkins, E.B., Cockreham, C., Wu, D., Mao, Y., Fischer, T.B., Stokes, M.R. and Xu, H., 2020b. Small-angle Neutron Scattering (SANS) Characterization of Clay-and Carbonate-rich Shale at Elevated Pressures. Energy & Fuels, https://doi.org/10.1021/acs.energyfuels.0c01009
Technical contacts: Hongwu Xu and Chelsea Neil (SANS); Hari Viswanathan and Qinjun Kang (Molecular simulations)
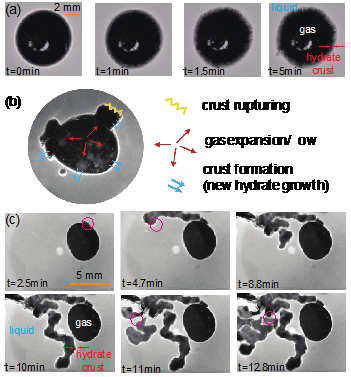
Experimental photographs taken in a high-pressure microfluidics system of the temporal evolution of escaping gas and hydrate formation: (a) a xenon gas bubble emerges at a leak site at high pressure (7.5 MPa or 1,080 psi). As the bubble grows, it is encased in a hydrate crust that temporarily halts its progress; (b) as internal pressure in the bubble increases, the crust eventually ruptures; (c) the process of rupturing and new hydrate formation results in the development of “crustal fingers” that encase a pathway allowing for gas migration with the hydrate stability zone.
Second study: Deep-sea methane migration mechanics unveiled
Los Alamos EES researchers also studied how methane is trapped and released in the deep sea. There are enormous quantities of methane contained in deep-sea sediments in the form of ice-like compounds called methane hydrate. This methane dwarfs the amount of oil and gas contained in conventional reservoirs.
In oceans across the world, there are numerous observations showing escape of methane gas from the sea floor—raising concerns about the potential impacts of methane hydrate stability on climate change. These observations have puzzled and worried scientists because the methane gas is escaping from sediments where the hydrate phase is stable and the methane should therefore be immobile.
Los Alamos researchers developed an experiment system in the high-pressure microfluidics laboratory to investigate gas migration under hydrate stable conditions and developed a new theory with collaborators based on numerical simulations that explains these troubling observations.
The EES team worked with xenon gas, which forms a hydrate phase directly analogous to methane hydrate, and observed gas migration occurring at 25 oC and 7.5 MPa pressure (1,090 psi). These conditions showed that as gas emerges it is initially trapped by a crust of hydrate. However, internal pressure in the leak soon ruptures the crust. This leads to a dynamic process of crust rupture and renewed hydrate growth that generates a hydrate-crust-encased finger that provides a pathway for migration of the gas.
Numerical modeling based on the experimental results was able to reproduce crustal finger formation as a result of the competition between flow of gas and crust formation. The crust acts as a transport barrier that prevents complete solidification of the gas to hydrate. The thin crust cannot support increasing internal pressure and ruptures releasing gas. Depending on the rate of crust formation, the gas is quickly encased in hydrate crust, and a propagating, branching crustal finger of hydrate is formed. The combination of experiment, simulation, and theory explains observations made at the sea floor. The crustal fingers allow hydrate and gas to exist at the same time in depths of ocean sediments where hydrate should be the stable phase. Simultaneously, the crustal fingers provide a conduit for gas migration. Growth of the crustal fingers eventually provides a mechanism for methane to migrate from deep-sea sediments onto the sea floor.
Funding and mission
The work at Los Alamos was funded by the Department of Energy’s Office of Science Basic Energy Sciences Program (LANLE3W1). The work supports the Laboratory’s Energy Security mission area and the Complex Natural and Engineered Systems capability pillars.
Reference
Fu, X., Jiménez-Martínez, J., Nguyen, T. P., Carey, J. W., Viswanathan, H., Cueto-Felgueroso, L., and Juanes, R. (2020). Crustal fingering facilitates free-gas methane migration through the hydrate stability zone. Proceedings of the National Academy of Sciences. https://doi.org/10.1073/pnas.2011064117
Technical contacts: J. William Carey and Hari S. Viswanathan
Proposal Call
Laboratory Directed Research and Development proposal calls due soon
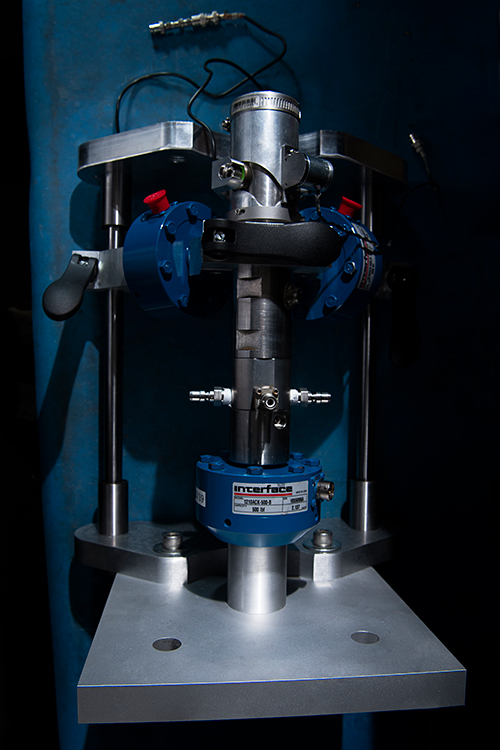
The restartable rocket motor represents an LDRD success story. This technology received program funding and an R&D 100 Award.
The Los Alamos Laboratory Directed Research and Development (LDRD) program is requesting proposals in three categories: fiscal year (FY) 2022 directed research, FY22 exploratory research, and FY21 exploratory research seedling pilot.
Los Alamos National Laboratory is entrusted with some of the world’s most urgent, complex problems. The LDRD Program, established by Congress, is one of the Laboratory’s essential investment strategies to enable agile responses to national security challenges and advance the frontiers of science, technology, and engineering.
Pre-proposals for directed research are due Feb. 4, 2021. Pre-proposals for exploratory research, including the seedling pilot, are due Feb. 8–Feb. 11, 2021, staggered by technical category.
- Feb. 8: High-Energy-Density Matter, Plasma, Fluids, and Beams (HPFB) and Quarks to the Cosmos (QTC)
- Feb. 9: Advanced Materials Science and Engineering (AMSE), Atomic, Molecular, Quantum and Optical Science (AMQOS), and Emergent Materials Behavior (EMB)
- Feb. 10: Biological Sciences (BIOS) and Chemical Sciences (CHEM)
- Feb. 11: Computational Methods and Computer Science (CMCS), Data Science and Mathematics (DSM), and Earth and Space Sciences (ESS)
More information about the proposal calls can be found on the LDRD site. Pre-proposals must be uploaded to the Q system for consideration. Classified submissions will be considered; however, the submission process is slightly different. Guidance can be found on the LDRD site.
The Los Alamos LDRD program encourages high-risk, high-reward research, creativity, and genius. Many LDRD-funded projects have gone on to receive program funding, R&D 100 awards, and other high-level accolades.
Theoretical
Material advances: 2D plasmonic nanostructures to harness quantum light
New research at the crossroads of plasmonics, quantum optics, and 2D materials shines light on an exotic quantum state—an entangled two-photon state—providing a foundation for novel technologies in nanophotonics. Some of these new technologies include active control of single-to-multiband emission spectra for sensing and spectroscopy functionalities, rapid generation of two-photon entangled states for quantum cryptography, and opportunities to develop novel infrared two-quanta sources with high quantum efficiencies.
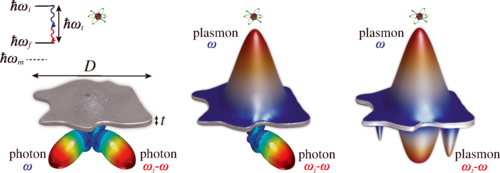
This schematic of the 2D plasmonic nanostructure illustrates the pathways for generating two-quanta states, which can be entangled in time-energy, linear momentum, or angular momentum.
“We found that two-dimensional plasmonic nanostructures are an ideal material platform to harness two-quanta emission processes in single quantum emitters,” says Wilton Kort-Kamp of Physics of Condensed Matter and Complex Systems (T-4).
Although entangled two-photon states are exotic, they have been previously achieved in nonlinear crystals, semiconductors, and quantum dots. However, efficiently achieving these states using individual emitters is difficult because their generation rate is much slower than competing single-photon processes. In a recent Los Alamos-led study, Kort-Kamp and colleagues were able to theoretically overcome this challenge and achieve “giant” generation of two-photon states from a single emitter.
“Our photon-generation rate was several orders of magnitude larger than previous techniques, and that was actually due to ultra-confined bright surface plasmons that scatter at the border of 2D nanostructures and leak to the far-field as photons,” Kort-Kamp says.
These surface plasmons were key to assisting ultra-high photon generation via two-quanta transitions in nearby single quantum emitters. Surface plasmons are electromagnetic excitations that exist at the interface between metallic and dielectric materials. In extended materials, they typically dissipate into heat after propagating a few dozen nanometers, and outcoupling them to generate photons via defect engineering is often challenging. On the other hand, finite-size plasmonic systems provide a path for the plasmons to leak as photons via the nanostructure’s border, which the researchers have explored to achieve fast two-photon emission.
The researchers state that their findings can be experimentally verified using ultra-thin plasmonic nanostructures, multi-level quantum dots, state-of-the-art photo coincidence, and time-resolved fluorescence spectroscopy. They are investigating structural and material designs for optimal maximization of the effect in future experimental demonstrations. Additionally, the researchers are studying other plasmonic mechanisms to enhance quantum photon generation and effects of plasmonic nanostructures in other exotic quantum processes.
Funding and mission
This research was funded by a LANL LDRD award. The work supports the Laboratory’s Energy Security mission area and the Materials for the Future capability pillar.
Reference: Y. Muniz, A. Manjavacas, C. Farina, D. A. R. Dalvit, and W. J. M. Kort-Kamp. “Two-Photon Spontaneous Emission in Atomically Thin Plasmonic Nanostructures.” Phys. Rev. Lett. 125, 033601 (2020). https://journals.aps.org/prl/abstract/10.1103/PhysRevLett.125.033601
Technical contact: Wilton Kort-Kamp