Science Highlights, September 2, 2015
Educational Outreach
Nuclear Safeguards and Security Summer School trains the next generation of experts
Materials Physics and Applications
Long-lived electron spin relaxation and spin coherence in atomically-thin semiconductors
Materials Science and Technology
Implementing statistics to examine x-ray computed tomography data of polymer foams
Chemistry
New chelating agents for bismuth isotopes developed for cancer therapy
The Laboratory’s Isotope Production team produces radioisotopes for use in research and medicine. The team has developed a promising new way of using an important radioactive isotope of bismuth (Bi) in cancer therapy. Nuclear Medicine and Biology has published their findings.
High-energy alpha (α)-particles emitted by the decay of radioactive isotopes can be harnessed to destroy malignant cells. This therapeutic strategy, known as α-therapy, is the subject of much study. The FDA recently approved the α-emitter 223RaCl2 for the treatment of bone metastases arising from a particular type of cancer. The short range (several cell diameters) and high linear energy transfer (approximately 100 keV/μm) of emitted α-particles suggests their use in the treatment of micro-metastatic disease, where the decay energy could be limited to the targeted cells. However, the limited availability of α-emitting radionuclides with appropriate physical properties for therapy hinders their development for clinical application. Binding these radioisotopes to chelating agents (molecules that can form several bonds to a metal ion) and appropriate biological targeting vectors to direct the complexes to the malignant cells present technical challenges. Better chelation and targeting methods for the radioisotopes could achieve significant medical benefits for therapy.
Bismuth-213 (213Bi) has potential for targeted α-therapy. The short half-life is suitable for use with small-molecule and peptide-based targeting agents. Its half-life and small decay chain minimize toxic side effects that may arise from long-lived daughter nuclides. 213Bi can be obtained from a commercially available generator system comprised of its longer-lived parent actinium-225 (225Ac). Some clinical trials have demonstrated the utility of 213Bi for cancer treatment. However, challenges associated with the aqueous chemistry of the metal ion and binding Bi3+ using ligands (ions or molecules that bond to a central metal ion) have hampered its use in targeted α-therapy.
Figure 1. Structures and abbreviated names of ligands investigated.
The Los Alamos team examined a new series of nitrogen-rich macrocyclic ligands (depicted as L in Figure 1) and explored its coordination chemistry with Bi3+. The researchers compared the binding of Bi3+ provided by the new ligands with that of some commonly used ligands shown in Figure 1 (top row).
Unlike other commercially available ligands such as DOTA, these new ligands are selective for Bi3 + even in the presence of the parent radioisotope Ac3+. Density functional theory (DFT) calculations corroborate the experimentally observed selectivity of these ligands for Bi3 + compared with Ac3+. This selectivity is important to minimize detrimental effects of 225Ac/213Bi generator breakthrough that could lead to inadvertent patient dosing with small quantities of long-lived 225Ac.

Figure 2. Crystal structure of Bi3+ bound to Lpy; the chemical formula is denoted as [Bi(Lpy)(OTf)](OTf)2].
The researchers found that subtle electronic modifications across the series of ligands cause significant effects on the solution behavior of their Bi3 + complexes. Among the four new ligands tested, Lpy (Figure 2) exhibits optimal Bi3 +-binding kinetics and complex stability. The investigators concluded that this class of nitrogen-rich ligands might be used for the rapid, selective, and stable chelation of radiobismuth for targeted α-therapy. The information gained from the research provides insight for the rational design of ligands for therapeutic radioisotopes.
Reference: “Evaluation of Nitrogen-Rich Macrocyclic Ligands for the Chelation of Therapeutic Bismuth Radioisotopes,” Nuclear Medicine and Biology 42, 428 (2015); doi:10.1016/j.nucmedbio.2014.12.007 Authors: J. J. Wilson, M. Ferrier, V. Radchenko, J. R.
Maassen, J. W. Engle, F. M. Nortier, and M. E. Fassbender (Inorganic, Isotope and Actinide Chemistry, C-IIAC); E. R. Batista and R. L. Martin (Physics and Chemistry of Materials, T-1); K. D. John and E. R. Birnbaum (Office of Science, SPO-SC).
The DOE, Office of Science Isotope Development and Production for Research and Applications subprogram and the Heavy Element Chemistry Program of DOE Basic Energy Sciences funded different aspects of the work. The Laboratory Directed Research and Development (LDRD) program sponsored a postdoctoral fellowship, and some researchers received Seaborg Institute Fellowships. The work supports the Lab’s Materials for the Future area through the development of isotopes for radiation therapy treatment. Technical Contact: Eva Birnbaum
Earth and Environmental Sciences
Isotopic signals of nitrate sources in tundra soils and permafrost
Los Alamos investigators and collaborators from Oak Ridge National Laboratory and the Permafrost Laboratory at the University of Alaska – Fairbanks have identified isotopic signatures of different nitrate (NO3-) sources in Arctic tundra soils and permafrost. The Journal of Geophysical Research – Biogeosciences published the findings. This work has important implications for understanding changes in nitrate biogeochemistry and the subsequent consequences of landscape evolution associated with warming and permafrost thaw.
Permafrost thaw and the resulting landscape reorganization could significantly alter the nitrogen cycle. This would affect soil organic matter decomposition rates and vegetation productivity with feedbacks to the coupled carbon cycle. Therefore, investigators characterized isotopic signals of nitrate sources and transformations to better understand the nitrogen cycle in tundra settings and to assess the use of these signals as potential tracers of hydrologic transport of nitrogen. The team used the dual isotope measurement of nitrogen and oxygen isotopes in NO3- to investigate atmospheric versus microbial sources. There are two different stable isotopes of nitrogen (14N and 15N) and three different isotopes of oxygen (16O, 17O, and 18O).
The team conducted the study at the Barrow Environmental Observatory on Alaska’s North Slope. This low gradient area consists of seasonally thawed active layer soil overlying thick, continuous permafrost. The relief is generally flat, though microtopography exists in the form of polygonal terrain. Most of the soils are saturated and reducing except for the centers of high-centered polygons, which are generally above the water table and are more oxic (Figure 3). Nitrate, an oxidized species of nitrogen, tends to accumulate in the raised centers of these high-centered polygons.

Figure 3. Isotopic composition of nitrate in massive ice, textural ice, and the unsaturated active layer of the centers of high-centered polygons.
The primary sources of nitrate in the Barrow area are atmospheric nitrate deposition and nitrate formed from microbial mineralization and nitrification of organic matter. These sources have distinct nitrogen and oxygen isotopic signatures. The nitrate found in the drier soils of high-centered polygons is clearly microbial in origin. The lack of an atmospheric nitrate signal does not preclude atmospheric nitrate as an important source of “new” nitrogen to the system. Rather, the results show that atmospheric nitrate is rapidly consumed into biomass, which is then subject to decomposition to form microbial nitrate. Isotopic measurements could potentially be used to trace local transport of microbial nitrate from high-centered polygons (and probably from rims of low-centered polygons) into surrounding troughs. On a larger scale, this technique may be useful to trace microbial nitrate from large areas of high-centered polygons into surrounding lower terrain. The persistence of this signal should be evaluated. Outside of the snowmelt period, any nitrate transported laterally may be rapidly consumed by plants and microbes. Isotopes could play a role in elucidating this process because microbial denitrification imparts a distinct isotopic signature. Most saturated areas in these studies have yielded insufficient nitrate for isotopic analysis. Therefore, the team is developing techniques to concentrate nitrate collected over time from saturated settings.

Figure 4. Approximate 18O versus 15N of nitrate in snowmelt, active layer pore water from high-centered polygons, and permafrost. Permafrost ice is separated into massive (e.g. ice wedge) and surrounding textural ice in this figure. Based on records from Greenland ice cores, pre-industrial atmospheric nitrate may have had a higher 15N.
Plant-available nitrogen stored in permafrost has been called a “frozen feast.” The dissolved inorganic nitrogen that could be quickly assimilated by plants and microbes upon thaw represents “fast food”, and soil organic matter that must first undergo mineralization represents “slow food”. Therefore, investigators also studied the isotopic composition of permafrost nitrate (“fast food”) that could potentially be mobilized to the active layer upon permafrost thaw. The team discovered that the isotopic composition of this nitrate varied between massive ice (ice wedges found beneath the troughs of high-centered polygons and beneath the rims of low-centered polygons) and textural ice (ice in the pores of frozen sediment surrounding massive ice). Nitrate from massive ice had a higher nitrate oxygen isotope signature, indicative of a greater component of atmospherically-derived nitrate. The oxygen isotope signature of the ice itself suggests a winter source of precipitation. These results are consistent with a model in which soil cracking and late winter infiltration of snowmelt, containing accumulated atmospheric nitrate deposits, leads to the formation of massive ice wedges. Conversely, ice and nitrate oxygen isotope signatures in surrounding sediment-rich textural ice are consistent with a greater proportion of summer precipitation and nitrate formed by microbial processes. Moreover, the isotopic composition of nitrate from both types of permafrost is distinct from that found in drier active layer soils found in the centers of high-centered polygons. While there is significant variation in this isotopic signal, upon large-scale melt such isotopic variation would be averaged out to provide a signature at the base of newly thickened active layer soils diagnostic of permafrost thaw. The team suggests that the persistence of this signal both in-situ and with lateral transport should be further investigated in areas where significant active permafrost thaw is occurring. Studies to begin this summer in the warmer Seward Peninsula of Alaska, where permafrost is discontinuous and rapidly degrading, will help test this hypothesis.
Reference: “Isotopic Identification of Soil and Permafrost Nitrate Sources in an Arctic Tundra Ecosystem,” Journal of Geophysical Research – Biogeosciences, doi: 10.1002/2014JG002883. Authors: J. M. Heikoop, H. M. Throckmorton, B. D. Newman, G. B. Perkins, and C. J. Wilson (Earth System Observations, EES-14); C. M. Iversen, T. Roy Chowdhury, D. E. Graham, R. J. Norby, and S. D. Wullschleger (Oak Ridge National Laboratory); and V. Romanovsky (University of Alaska – Fairbanks).
The DOE Office of Biological and Environmental Research, Next Generation Ecosystem Experiment (NGEE)-Arctic funded the research, which supports the Lab’s Global Security mission area and the Science of Signatures science pillar via studies of indicators to determine the mechanism and effects of climate change. Technical contact: Jeff HeikoopEducational Outreach
Nuclear Safeguards and Security Summer School trains the next generation of experts

Photo. (Left to right): Peter Santi (Safeguards and Technology, NEN-1) instructs Stacy Queern (Washington University, St. Louis), Jason Richards (University of Nevada, Las Vegas), and Athena Gallardo (University of Nevada, Las Vegas) on active neutron nondestructive assay measurement techniques for uranium items.
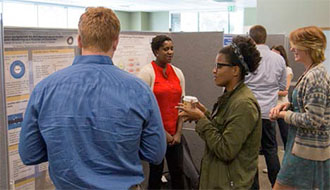
Photo. Participants discuss their research during the poster session.
The Nuclear Science and Security Consortium (NSSC), Sandia National Laboratories (SNL), and LANL held a joint Nuclear Safeguards and Security Summer School from August 3-12. The school’s purpose was to train the next generation of experts in nuclear security. The program featured a five-day module at Los Alamos followed by a three-day module at SNL. Twenty-four students from seven universities participated.
The Los Alamos Nuclear Safeguards component provided an introduction to the nondestructive assay (NDA) of uranium and plutonium-bearing materials using gamma ray and neutron measurement techniques. Students had hands-on training using measurement techniques to quantify and characterize items containing Special Nuclear Materials including uranium enrichment measurements, plutonium isotopic measurements, active neutron coincidence counting of uranium, and passive neutron coincidence counting of plutonium. Los Alamos presenters included Mark Croce, David Miko, Karen Miller, Peter Santi, and Martin Swinhoe (Safeguards and Technology, NEN-1); Raspberry Simpson (Neutron Science and Technology, P-23); and Peter Karplus.
NSSC students participated in hands-on exercises at Los Alamos involving nondestructive assay measurements of uranium- and plutonium-bearing items as well as a mock material verification exercise. The students discussed their ongoing research projects with Lab staff and NSSC campus-based faculty during a welcome event and poster session. Organizers designed the session to catalyze collaboration between Lab and university researchers and highlight the research of the students in radiochemistry, nuclear physics, nuclear engineering, instrumentation, and national security policy. LANL gave students tours of LANSCE, space-qualified flight hardware laboratory spaces, the high-explosive testing area, and the National High Magnetic Field Laboratory (NHMFL).
The NSSC is a consortium of universities led by University of California – Berkeley. NNSA’s Office of Defense Nuclear Nonproliferation R&D funds the consortium to support the nation’s nuclear nonproliferation mission through the training and education of experts in the nuclear security field. Other NSSC universities: Michigan State University, University of California – Davis, University of California – Irvine, University of California – San Diego, University of Nevada – Las Vegas, and Washington University – St. Louis. Students in the consortium can collaborate with researchers at four DOE national laboratories – Los Alamos, Lawrence Berkeley, Lawrence Livermore, and Sandia. Michael Rabin (Space and Remote Sensing, ISR-2) is the LANL point of contact for the NSSC. The work supports the Lab’s Global Security mission area and the Science of Signatures science pillar through LANL’s nuclear nonproliferation activities. Technical contact: Michael Rabin
Materials Physics and Applications
Long-lived electron spin relaxation and spin coherence in atomically-thin semiconductors
Researchers at the National High Magnetic Field Laboratory in Los Alamos (NHMFL-LANL) have directly measured the very long spin lifetimes in the two-dimensional (2-D) “Dirac semiconductors” molybdenum disulfide (MoS2) and tungsten disulfide (WS2). The results reveal the underlying physics governing the coupled spin-valley dynamics of these materials and demonstrate the potential for spin- and valley-based logic devices and quantum information. Nature Physics published the research.
The recently discovered monolayer MoS2 and related atomically-thin transition metal dichalcogenides are analogous to graphene in that they are 2-D materials with hexagonal honeycomb structure. They display an extremely important difference: unlike graphene, transition metal dichalcogenides possess a semiconductor bandgap. This difference makes transition metal dichalcogenides useful for a variety of opto-electronic applications, including solar, light-emitting diodes, and semiconductor electronics. Much recent interest in these new monolayer semiconductors stems from the fact that electrons in these materials possess a “valley pseudospin” degree of freedom that is analogous to an ordinary electron’s spin degree of freedom. “Valley” refers to the electron momentum: electrons in these 2-D materials can occupy one of two possible momentum states (the K and K’ points of the material’s Brillouin zone; see Figure 5). This valley degree of freedom can be directly and selectively addressed with polarized light in these materials. In addition to the real electron spin, information could also be encoded in the “valley pseudospin”, i.e. whether the electron resides in the K or K’ valley of the materials’ hexagonal Brillouin zone (or, a quantum superposition of K and K’). Although a robust valley degree of freedom in transition metal dichalcogenides has been inferred from photoluminescence experiments, the exploration of spin-valley coupled dynamics and decoherence of resident electrons in electron-doped material is at a very early stage.
Figure 5. a) 2-D hexagonal lattice of MoS2, the first Brillouin zone, and the valley-contrasting optical selection rules present in these 2-D materials. The interband transition in the K/K’ valley couples to right/left- circularly polarized light only. b) Time-resolved Kerr rotation data (using pulsed pump and probe lasers) directly reveals very long electron relaxation dynamics in MoS2 at different applied magnetic fields.
The team, led by Laboratory scientists collaborating with materials scientists at Rice University, directly probed the coupled spin and valley dynamics in electron-doped monolayer MoS2 using techniques based on optically-induced Kerr rotation spectroscopy. In contrast to photoluminescence studies, these techniques directly probe the spin and valley polarizations of electrons alone (rather than excitons), which can persist long after recombination with photoexcited holes. The researchers used Kerr-effect methods to directly and unambiguously measure very long intrinsic electron spin relaxation timescales of approximately 3 ns (Figure 5), which are 2-3 orders of magnitude longer than the exciton recombination time. Measurements as a function of applied magnetic fields indicate that electrons “see” a strong effective magnetic field due to the large spin-orbit coupling in these 2-D materials, and that electrons undergo rapid intervalley scattering between the K and K’ valleys of the materials’ hexagonal Brillouin zone. A long-lived oscillatory signal observed at lower energies indicates that some electrons are localized and exhibit robust spin coherence. These studies provide direct insight into the intrinsic physics underpinning spin and valley dynamics of resident electrons in transition metal dichalcogenides. Observation of nanosecond long spin relaxation in transition metal dichalcogenides opens an alternative route for spintronic science and applications.
Reference: “Long-lived Nanosecond Spin Relaxation and Spin Coherence of Electrons in Monolayer MoS2 and WS2,” Nature Physics (2015) advance online publication, doi: 10.1038/nphys3419. Authors: Luyi Yang (Condensed Matter and Magnet Science, MPA-CMMS), Nikolai Sinitsyn (Physics of Condensed Matter and Complex Systems, T-4), Weibing Chen, Jiangtan Yuan, Jing Zhang, and Jun Lou (Rice University); and Scott Crooker (MPA-CMMS).
The Laboratory Research and Development (LDRD) program funded the Los Alamos work. The research benefited from the use of the NHMFL Pulsed Field Facility at LANL, one of three campuses of the National High Magnetic Field Laboratory, which is sponsored primarily by the National Science Foundation, Division of Materials Research, with additional funding from the State of Florida and the DOE. This research supports LANL’s Energy Security mission area and the Materials for the Future science pillar through the development of materials for semiconductor applications. Technical contacts: Luyi Yang and Scott Crooker.
Materials Science and Technology
Implementing statistics to examine x-ray computed tomography data of polymer foams
A statistically based data analysis technique called principal components analysis is helping Los Alamos researchers analyze and interpret large amounts of data when comparing polymer foam samples from nuclear weapons.
X-ray computed tomography of materials provides large three-dimensional (3-D) image data sets (i.e., tomograms), resolving both surface and subsurface features. Segmenting the tomograms for the void structure allows for various 3-D measurements using image-processing software. One common void measurement is equivalent diameter, which is the calculated diameter assuming the void volume constitutes a perfect sphere. However, for stochastic and irregular void structures, this singular metric is insufficient when comparing two or more polymer foam samples. Multiple descriptors are typically required, but more than three measurements can be difficult to interpret.
The team used principal component analysis to examine the data. Principal component analysis is commonly used for pattern recognition in experimental sciences and enables easy visualization of sample groupings based on several descriptors. The technique transforms N-dimensional data into a reduced number of dimensions, thereby capturing most of the data’s variance.
In this preliminary proof-of-concept study, Engineered Materials (MST-7) researchers examined 30 polymer foam samples punched from 5 separate locations (Locations A-E) of a larger weapons part. Fifteen (3 from each location) were collected from an unused part, and 15 separate samples were collected from a used part that had approximately 20 years of use. The team considered Location A-D samples as equivalent to one another, and they considered Location E samples as inequivalent to those from Locations A-D.
Figure 6 shows volume renderings of four samples. Voids are colored by equivalent diameter. The voids vary considerably, but it is difficult to differentiate each polymer foam sample from equivalent diameters alone. Therefore, the researchers used image-processing software to calculate void morphology and orientation for 15 separate void descriptors.
Figure 6. Volume renderings, derived from X-ray tomograms, of the void structure of four polymer foam parts. The voids are colored by their calculated equivalent diameters.
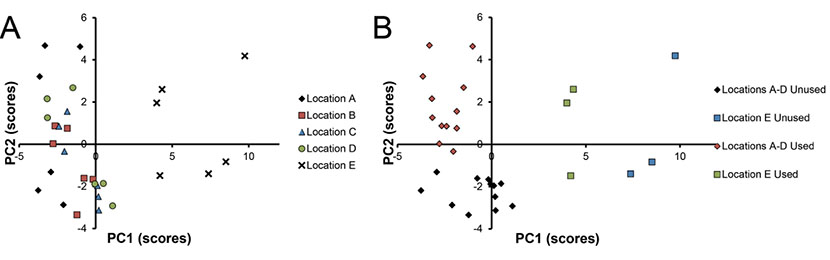
Figure 7. Principal Component 1 (PC1) compared with Principal Component 2 (PC2). A) Samples from Locations A, B, C, and D (black diamonds, red squares, blue triangles and green circles, respectively) group well with one another, whereas samples from Location E (black crosses) are loosely grouped separately, indicating large differences in void morphology and orientation. B) Data are colored by their history (Locations A-D Unused, black diamonds; Locations A-D Unused, red diamonds; Location E Unused, blue squares; Location E Used, green squares).
A similar concurrent analysis of polymer foam stress-strain data indicates that principal components analysis could also distinguish mechanical performance of new and used materials. The team will concentrate on variable selection (i.e., down-selecting 3D measurements), incorporating void measurements at specific compressive strains, and developing a multivariate model of polymer foam performance.
The team includes: Nikolaus L. Cordes, Zachary Smith, Kevin Henderson, and Brian M. Patterson (MST-7). NNSA’s Surveillance and Directed Stockpile Work programs funded the work, which supports the Lab’s Nuclear Deterrence mission area and the Information, Science, and Technologies science pillar. Technical contact: Nikolaus L. CordesPhysics
Lab participates in three DOE Advanced Research Projects Agency-Energy studies
The DOE Advanced Research Projects Agency-Energy (ARPA-E) has announced $30 million in funding for nine groundbreaking new projects aimed at developing prototype technologies to explore new pathways for fusion power. ARPA-E advances high-potential, high-impact energy technologies that are too early for private-sector investment. ARPA-E awardees are developing entirely new ways to generate, store, and use energy. The new projects are funded through ARPA-E’s Accelerating Low-cost Plasma Heating and Assembly (ALPHA) program, which seeks to develop low-cost fusion energy technology solutions. Three of the projects involve Los Alamos researchers and partners as described in the following paragraphs. Details on ALPHA’s nine projects: http://arpa-e.energy.gov/sites/default/files/documents/files/ALPHA Project Descriptions_FINAL.pdf.
The Los Alamos National Laboratory projects are the following:
Spherically Imploding Plasma Liners as a Standoff Magneto-Inertial-Fusion Driver
Plasma Physics (P-24) researchers in collaboration with HyperV Technologies Corporation and a multi-institutional team will focus on magneto-inertial fusion (MIF) concepts with reactor-friendly attributes. The investigators will develop a plasma-liner driver formed by merging supersonic plasma jets produced by an array of coaxial plasma guns.
Magneto-inertial fusion uses a magnetic field to reduce thermal transport rates and enhance alpha (a)-particle – a product of deuterium/tritium fusion – energy deposition in imploded fusing fuel. These attributes create the possibility of igniting the fusion fuel in a pulsed manner at ion densities intermediate between those of mainline concepts of magnetic confinement fusion (MCF) and inertial confinement fusion (ICF). This intermediate-density regime represents a lower cost path to fusion development because MIF minimizes the combination of required stored energy and heating power, and thus the capital cost of a “fusion-driver” facility, to ignite fusion fuel. An energy break-even class facility for MIG should cost approximately $100 M compared with the multi-billion-dollar costs of break-even class MCF and ICF facilities. Sandia National Laboratories’ Magnetized Liner Inertial Fusion experiments demonstrated MIF proof of concept when it reached fusion-relevant conditions via cylindrical liner compression of laser-heated, magnetized deuterium fuel. However, the significant hardware destruction in each shot (i.e., the liner and transmission line connections) presents significant challenges to attain a reasonable R&D pace and to achieve the repetitively fired system needed for economic fusion power.
Therefore, the team will focus on a plasma liner formed by a spherical array of supersonic plasma jets launched by innovative coaxial plasma guns to compress a magnetized target to fusion conditions. This technique, known as plasma-jet-driven MIF, eliminates repetitive hardware destruction and allows for approximately 1 Hz operation – required to realize an economic fusion reactor with modest yield/shot (e.g., less than 500 MJ), which is highly desirable to simplify the substantial reactor-engineering challenges.
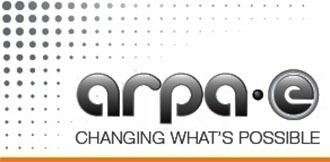
Key objectives of the new project are to:
- Advance the engineering reliability, operability, and precision of the coaxial guns.
- Make the first demonstration of the formation of a spherically imploding plasma liner via up to 60 supersonic plasma jets.
- Obtain crucial empirical data on the scaling of peak liner ram pressure and liner uniformity (the two key metrics for eventually compressing a magnetized target plasma to fusion-relevant pressures of approximately 100 Mbar).
Los Alamos researchers are Scott Hsu and John Dunn (P-24). Hsu is the Principal Investigator. The project supports the Laboratory’s Energy Security mission area and Nuclear and Particle Futures science pillar through studies of lower-cost fusion-energy development. Technical contact: Scott Hsu
Stabilized Liner Compressor (SLC) for Low-Cost Fusion


Figure 8. (Left): Schematic of the innovative shaped coaxial guns, designed and built by HyperV Technologies Corporation, which will be further developed with a gaseous injection system to form and launch plasma jets. (Right): Schematic of 60 plasma jets merging to form a spherically imploding plasma liner in the 9-foot-diameter vacuum chamber of P-24’s Plasma Liner Experiment.
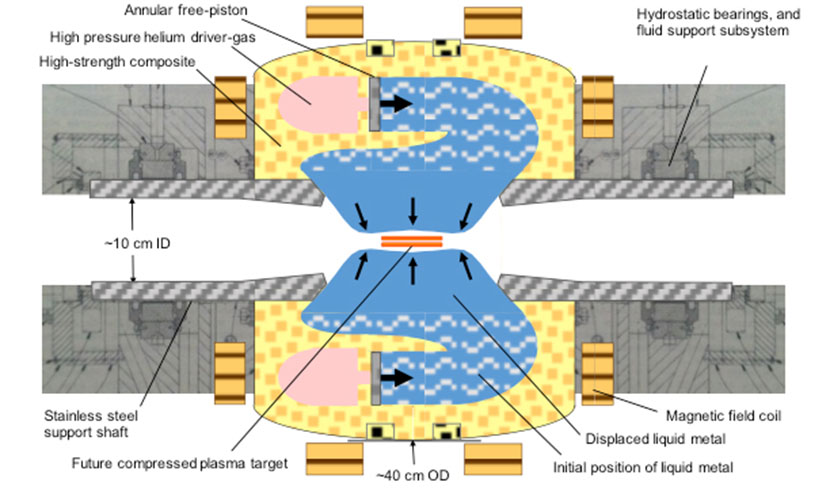
Figure 9. The Stabilized Liner Compressor system uses high-pressure gas and a free-piston to implode a liquid metal liner onto trapped magnetic flux to achieve controlled fusion at very high magnetic fields (approximately 100 T).
Los Alamos researchers will design and build a flux compression magnet system capable of producing magnetic fields of the order 50-100 tesla. Chuck Mielke (Condensed Matter and Magnet Science, MPA-CMMS) is the LANL project leader. Laboratory researchers include Peter Turchi (Physics, P-DO), Doan Nguyen, Mark Hinrichs, James Michel, Jason Lucero, Mike Pacheco, and Chuck Mielke (MPA-CMMS).
The work supports the Laboratory’s Energy Security mission area and Materials for the Future science pillar by leveraging advances in materials to develop a revolutionary high magnetic field capability to aid for fusion energy generation. Technical contact: Chuck Mielke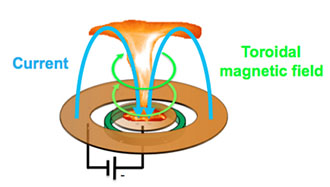
Figure 10. Schematic shows the high speed plasma outflow (red color) driven by the magnetic fields (green) and the current (blue) drawn from a capacitor bank. Such high speed plasma flows will collide with other plasma clouds and/or walls and induce strong compressional heating. First principle studies of such dynamics will provide insight to understand plasma-liner implosions.
The California Institute of Technology and Los Alamos National Laboratory will investigate collisions of plasma jets and targets over a wide range of parameters to characterize the scaling of adiabatic heating and compression of liner-driven magnetized target fusion plasmas. The team will propel fast, magnetized plasma jets into stationary heavy gases or metal walls. The resulting collision, which is equivalent to a fast heavy gas or metal liner impacting a stationary magnetized target in a shifted reference frame, will enable the non-destructive and rapid investigation of physical phenomena and scaling laws governing the degree of adiabaticity of liner implosions. This study will provide critical information on the interactions and limitations for a variety of possible driver and plasma target combinations being developed across the ALPHA program portfolio.
Hui Li (Nuclear and Particle Physics, Astrophysics and Cosmology, T-2) and Shengtai Li (Applied Mathematics and Plasma Physics, T-5) will perform multi-dimensional magnetohydrodynamic and kinetic plasma simulations of the experiments to be carried out at the California Institute of Technology. The Los Alamos researchers will examine the dynamics of plasma compression to obtain information regarding the energy loss mechanisms during compression and ways to mitigate such losses. Hui Li is the lead Los Alamos scientist on the project.
This research supports the Laboratory’s Energy Security mission area and the Information, Science, and Technology and Nuclear and Particle Futures science pillars by providing first-principle understanding of compression of multi-dimensional plasma dynamics. Technical contact: Hui Li
Theoretical
Rare noncoding point mutations associated with schizophrenia and bipolar disorder

Figure 11. Langevin Dynamics simulations, based on the Extended Peyrard-Bishop-Dauxois nonlinear model of DNA, quantify the propensity for local bubble-formation - with and without the presence of a single point mutation in the flanking of the transcription factor YY1’s binding motif. This change in DNA breathing dynamics affects the binding of YY1 to DNA, which in turn influences the expression of MIR137/MIR2682, two microRNA genes implicated in schizophrenia and bipolar disorder. Sequence containing the risk allele A in close proximity to YY1 binding site predicts weak DNA breathing activity (top), in contrast to the much stronger breathing potential of the sequence with the major allele T (bottom). The white horizontal lines mark the single nucleotide polymorphism sites. The YY1 binding sequence ending at the single nucleotide polymorphism site is shown below the single nucleotide polymorphism line.
The American Journal of Human Genetics published a report examining the effect on DNA breathing dynamics and gene expression of single base pair variations [single nucleotide polymorphisms (SNPs)] implicated in schizophrenia and bipolar disorder. Los Alamos researchers Boian S. Alexandrov (Physics and Chemistry of Materials, T-1) and Alan R. Bishop (Science, Technology and Engineering, PADSTE) participated in the international team that conducted the study identifying rare risk variants and how the variants could confer risk for schizophrenia and bipolar disorder. Nature Index listed the paper as one of 14 significant papers in biology that LANL produced during the period of June 2014 to May 2015.
In the living cell, the double-stranded DNA molecule experiences thermal motions that induce spontaneous openings and re-closings of the double helix known as “DNA breathing” or “DNA bubbles.” The propensity for breathing is interrelated with the DNA sequence and local flexibility of the molecule and plays a key role in the gene expression. The gene expression controls the production of biomolecules necessary for the cell. Transcription factor proteins influence gene expression via binding to specific DNA segments (motifs) positioned at different regulatory locations in the genome. DNA breathing can regulate DNA-protein binding. This regulation involves both the motif and its flanking sequence. In principle, suppressing the binding of a specific transcription factor, genomic mutations, and variants that compromise local bubble patterns may suppress gene expression and may enhance the risk of a particular genetic disease. A disruption of biological function (e.g., suppressed transcription) may be caused by direct changes of the binding motif but also by long-range changes of the flanking of the motif. Changes of the nucleotide sequence could lead to a similar impairment in the breathing dynamics. Thus, seemingly inconspicuous variations in DNA sequence may result in disease onset or progression.
The team examined the effect of single base pair variations on DNA breathing dynamics and gene expression (Figure 11). These variations are implicated in schizophrenia and bipolar disorder. Schizophrenia genome-wide association studies (GWAS) had previously identified common risk variants in more than 100 susceptibility loci, but the contribution and functionality of rare variants at these loci were largely unexplored. In the paper, the researchers aimed to identify rare risk variants of strong effect.
The study’s results suggest the existence of rare functional noncoding schizophrenia and bipolar disorder risk variants at the MIR137 locus, possibly decreasing MIR137 expression by a nonlocal interaction between single nucleotide polymorphism in the flanking of the binding motif of the YY1 transcription factor and DNA breathing. For schizophrenia there had been no previous report of a single rare variant showing genome-wide significant association, even in recent large-scale exome-sequencing studies. Rare functional variants identified at genome-wide association studies-implicated loci explain additional genetic risk and could also provide unparalleled direct links to causal variants or mechanisms given their relatively larger effects compared with common risk variants at the same locus. The research also demonstrates an approach of analyzing rare noncoding variants based on a priori knowledge of directional functionality of a variant.
Reference: “A Rare Functional Noncoding Variant at the GWAS-Implicated MIR137/MIR2682 Locus Might Confer Risk to Schizophrenia and Bipolar Disorder,” The American Journal of Human Genetics 95, 744 (2014); doi: 10.1016/j.ajhg.2014.11.001. Authors: Boian S. Alexandrov (T-1), Alan R. Bishop (PADSTE), and researchers from NorthShore University HealthSystem, The University of Chicago, National Cancer Institute, University College London, Virginia Commonwealth University, Harvard Medical School, University of Southern California, Stanford University, Molecular Genetics of Schizophrenia collaboration, and the Genomic Psychiatric Cohort consortium.
A Laboratory Directed Research and Development (LDRD) Early Career grant funded Alexandrov’s simulations of DNA breathing dynamics. The work supports the Lab’s science pillar of Information, Science, and Technology for Integrative and Predictive Science via simulations to reveal how single base pair variations affect DNA breathing dynamics, gene expression, and disease. Technical contact: Boian Alexandrov