Science Highlights, March 4, 2015
Awards and Recognition
Materials Physics and Applications
Accessing optoelectronic properties in atomic layered semiconducting materials
Awards and Recognition
Sullivan Graham receives award and donates scholarships to Milenski and Brown

Jeri Sullivan
Jeri Sullivan Graham (Chemical Diagnostics and Engineering, C-CDE) received a Conservation Grant Award from the Climate Change Leadership Institute. The Institute, a nonprofit organization dedicated to addressing the climate crisis, gives annual community seed grant awards to encourage leadership, develops alliances and rewards responsibility in the advancement of climate stewardship. The Conservation Grant award focuses on energy, water, and climate. The Institute honored Sullivan Graham for providing expertise and time to develop the energy and water nexus initiative with the New Mexico Energy, Minerals and Natural Resources Department. She chose to “pay it forward” by using the grant award to support scholarships to two area college students, Helen Milenski and Chad Brown.
Sullivan Graham is a hydrogeologist and geochemist with 30 years of experience in environmental chemistry, ground-water hydrology, water treatment, systems modeling and field studies. She has focused on the chemistry and the treatment of water produced during oil and gas extraction, called “produced water”. Sullivan Graham serves as a science advisor to Secretary David Martin of the New Mexico Energy, Minerals, and Natural Resources Department, and she coordinates the Brackish Water working group under the Governor’s Drought Task Force. Sullivan Graham received a PhD in Earth and Environmental Science from the New Mexico Institute of Mining and Technology.
Helen Milenski and Chad Brown each received $500 Earth and Environmental Science scholarships sponsored by the Laboratory and the Climate Change Leadership Institute. The seed grant portion of Sullivan Graham’s award honored the two students in Northern New Mexico colleges by assisting with their higher education, research, and development in the fields of climate, energy, and/or water conservation. The students received their scholarships during a ceremony February 10 at the New Mexico Consortium facility in Los Alamos.
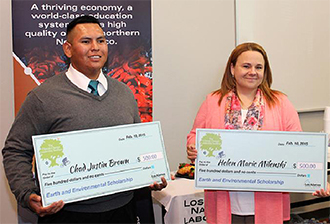
Photo. (Left to right): Chad Brown and Helen Milenski hold their scholarship checks.
Milenski is a non-traditional student attending the University of New Mexico-Los Alamos and pursuing a pre-engineering degree. Milenski has had an internship at the Laboratory. Her long-term goal is to pursue a mechanical engineering degree with a minor in material science at the New Mexico Institute of Mining and Technology. Brown is a freshman at Northern New Mexico University in Española pursuing a pre-forestry degree. He works at the Santa Clara Pueblo where he performs inventory and monitoring for their forestry department.
The scholarship applicants must have been full-time undergraduate students majoring in earth and environmental science or related fields at a college or university in Los Alamos, Mora, Rio Arriba, Sandoval, San Miguel, Santa Fe or Taos county. Technical contact: Jeri Sullivan Graham
Manard appointed Parliamentarian to the Society for Applied Spectroscopy
The Society for Applied Spectroscopy (SAS) has named Benjamin T. Manard, a current post-doctoral researcher in the Actinide analytical chemistry (C-AAC) group, to be a Parliamentarian on the executive committee of the society. The Parliamentarian serves as the expert on order and process for the committee. SAS was established over 50 years ago and is dedicated to “advance and disseminate knowledge and information concerning the art and science of spectroscopy and other allied sciences.” The society publishes the internationally recognized journal, Applied Spectroscopy.

Manard’s research as a graduate student, with Professor R. Kenneth Marcus at Clemson University, has provided him with many opportunities for extensive collaborations with DOE national laboratories. He developed a miniaturized plasma excitation/ionization source for elemental analysis. Manard worked at Lawrence Berkeley National Laboratory with Richard E. Russo (a Society for Applied Spectroscopy fellow) and at Pacific Northwest National Laboratory with David Koppenaal. In both DOE facilities, Manard fabricated and assessed a microplasma source for various analytical applications (laser ablation, mass spectrometry, and optical emission spectroscopy). His graduate research resulted in 11 peer reviewed journal publications and numerous conference presentations. These studies, publications, presentations at conferences, and enthusiasm for spectroscopy science led to this position with the Society for Applied Spectroscopy.
Currently, Manard works with Ning Xu in the plasma spectroscopy team of C-AAC at Los Alamos. His research focuses on material-at-risk (MAR) reduction methods for the Radiological Laboratory Utility Office Building (RLUOB) facility by employing automated actinide separation techniques (i.e. microfluidics) for trace elemental analysis by inductively coupled plasma (ICP-OES) techniques. He develops new ideas to study the microplasma source. This is an innovative approach to develop fieldable instrumentation for elemental and isotopic analyses, particularly for actinides. In February 2015, the Laboratory selected Manard for a Seaborg Fellowship, which will support the postdoc half-time for two years. Technical contact: Benjamin Manard
Bioscience
LANL helps BioRisk Management and Genomics Training Divisions open in Jordan
Helen Cui (Bioenergy and Biome Sciences, B-11) represented Los Alamos National Laboratory at the February 10 ribbon cutting ceremony and opening of the BioRisk Management and Genomics Training Divisions at the Princess Haya Biotechnology Center, Jordan University of Science and Technology, in Irbid, Jordan. For more than two years, Helen Cui and many Los Alamos colleagues, including Tracy Erkkila, Jose Olivares, Momchilo Vuyisich, Cheryl Gleasner, Chris Detter, Gary Resnick, Jennifer Harris, and Karen Hill, have contributed to this multi-national effort to build new capabilities in Jordan. The Los Alamos effort has received tremendous support from the senior management and program offices. The U.S. Cooperative Threat Reduction Programs at the Departments of State (BioSecurity Engagement Program) and Defense (Cooperative Bioengagement Program), the United Kingdom Global Partnership Programme, and the Canadian Ministry of Foreign Affairs Global Partnership Program have sponsored the establishment of the BioRisk Management and Genomics Training Center.
The new divisions will provide sustained biorisk management and genomics training and research that employ high standards in operations, safety and security, and research and development. This organization will serve the needs of Jordan and its Middle Eastern and North African neighbors and reduce health threats to the global community. The two new divisions are hosted by the Princess Haya Biotechnology Center, which was established in 2003 to serve as a center of excellence for biotechnology in Jordan. Los Alamos National Laboratory and Sandia National Laboratories have been key participants in the center development, including the facility renovation, instrument acquisition, technical staff training, and overseeing the set up of equipment and arrangement of operations.
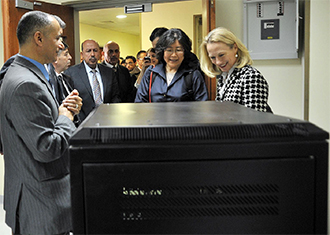
Photo. Helen Cui (center) explains the genomics center computer server features to the U.S. Ambassador, Ms. Alice Wells (right). Others in the photo (from the left): the Princess Haya Biotechnology Center Director, UK Ambassador (behind him), the Governor of Irbid, and the President and Vice President of the Jordan University of Science and Technology.
The U.S. Ambassador to Jordan (Ms. Alice G. Wells), British Ambassador to Jordan (Mr. Peter Millett), and Canadian Ambassador to Jordan (Mr. Bruno Saccomani) delivered congratulatory speeches at the opening ceremony. Professor Abdallah Malkawi, the President of the Jordan
University of Science and Technology, stated that the new divisions will: 1) open new research opportunities in genomics, 2) enhance the capacity for biorisk management and technological capabilities of laboratories in the developing world to combat infectious diseases, and 3) promote a sustainable culture of safety and security laboratory practice through the education and training of staff from the Middle Eastern North African region. Cui accepted the recognition plaque for LANL, along with Sandia National Laboratories and the donor program representatives, from Professor Malkawi. The governor of Irbid and more than 200 officials, academics, researchers and experts from Jordan, USA, and European countries attended the opening.
The U.S. Department of State and Department of Defense sponsor the LANL work, which supports the Laboratory’s Global Security mission area and the Science of Signatures science pillar. Technical contact: Helen Cui
Educational Outreach
Call for Nuclear Forensics Undergraduate Summer School applications
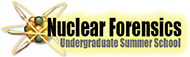
The Laboratory is a co-sponsor of the Nuclear Forensics Undergraduate Summer School, to be held June 15 through July 24 at Washington State University. The six-week summer school is in its sixth year. The school is designed to provide comprehensive, experimental, hands-on training in topics essential to nuclear forensics as a means of interesting students to pursue graduate studies in technical fields related to nuclear forensics. A primary goal of the school is ensuring an ongoing pipeline of American radiochemists because fewer young people are selecting radiochemistry as a career.

Photo. A student performs laboratory experiments during the course.
The course consists of a combination of laboratory work and lectures, many of which are given by experts in the nuclear forensics community from DOE National Laboratories and federal agencies. Coursework will cover major topics in nuclear and radiochemistry and the chemical and physical characterization of actinide-bearing materials. A field trip to Pacific Northwest National Laboratory will provide participants a first-hand view of an operational environment.
Undergraduate students who are U.S. citizens specializing in the physical sciences are encouraged to apply. Students will receive financial compensation for tuition, fees, housing, meals, and a stipend.
The application deadline is March 22, 2015. More information and application: http://pearl1.lanl.gov/external/nuclear-forensics/
The U.S. Department of Homeland Security, Los Alamos National Laboratory, Lawrence Livermore National Laboratory, Washington State University, University of Missouri, and the University of Nevada-Las Vegas sponsor the course. The summer school is the result of a contract LANL has with the Department of Homeland Security and is run by the Los Alamos branch of the Seaborg Institute. Stosh Kozimor (Inorganic, Isotope, and Actinide Chemistry, C-IIAC) is the primary organizer. Technical contacts at LANL: Susan Ramsay, Stosh Kozimor, and Kristen Norris
Experimental Capability Enhancement
Combined capabilities reveal how trees transport water
Los Alamos researchers have made the first simultaneous measurements of Ultra-Low-Field Nuclear Magnetic Resonance (ULF-NMR) and neutron imaging to visualize the movement of water in trees. Water use by trees is a key part of the hydrological process linking soil to climate and local weather. Despite decades of research and method development, non-destructive, in vivo measurements of water uptake and flow in trees are unavailable for field-based measurement. The lack of measurements limits progress towards understanding this important climate factor. Measurement challenges arise from the opacity of wood and the intricate nature of the water transport system, where opposing pressure gradients in adjacent tissues are responsible for water uptake from the soil and delivery of carbohydrates produced in the leaves.
To overcome these methodological challenges, LANL researchers from Earth System Observations, (EES-14), Applied Modern Physics (P-21), and Inorganic, Isotope and Actinide Chemistry (C-IIAC) have developed an ULF-NMR system that is suitable for use in ambient, outdoor environments to monitor long-term changes in the water contents of living trees non-destructively. Other NMR systems work in regulated temperatures, requiring a cooled magnet and maintaining the system at constant temperature. The ULF-NMR system does not need cooling. The journal Review of Scientific Instruments reported this system.
Reference: “Low-field Nuclear Magnetic Resonance for the In Vivo Study of Water Content in Trees,” Review of Scientific Instruments 85, 095110 (2014); doi.org/10.1063/1.4895648. Authors include: Jacob Yoder (Inorganic, Isotope and Actinide Chemistry, C-IIAC), Michael W. Malone and Michelle A. Espy (Applied Modern Physics, P-21), and Sanna Sevanto (Earth System Observations, EES-14).
The Laboratory scientists aimed to move toward field application by calibrating the NMR signal for water uptake by different tissues. Therefore, the team and colleagues from Non-Destructive Testing and Evaluation (AET-6), Materials Science in Radiation and Dynamics Extremes (MST-8), and LANSCE Weapons Physics (P-27) conducted simultaneous neutron radiography and ULF-NMR measurements of D2O (i.e. deuterium, also known as “heavy water”) uptake by living branches at the Los Alamos Neutron Science Center (LANSCE). The researchers used the 60-meter Lujan Center Flight Path 5 neutron beam line. Both NMR and neutron imaging allow differentiation between D2O and H2O (the more common isotope of water). Alternate watering of branches with these two water sources enabled the researchers to measure the flow rates with both systems simultaneously and compare them. The team also added a surfactant to the source water, thereby changing its surface tension and inducing bubble formation (cavitation) in the branch’s water conductive tissue. This condition induced failure of the conductive tissue to simulate drought conditions in nature.

Figure 1. (Left panel): Photograph of the NMR and neutron beam set-up. Neutrons enter at right, the NMR system surrounds the juniper branch, and the neutron detector is at left. (Right panel) Inset shows a neutron radiography image of the set-up. The branch is in the middle, the bars on both sides are the NMR shield, and the thickening on the branch is the NMR coil.
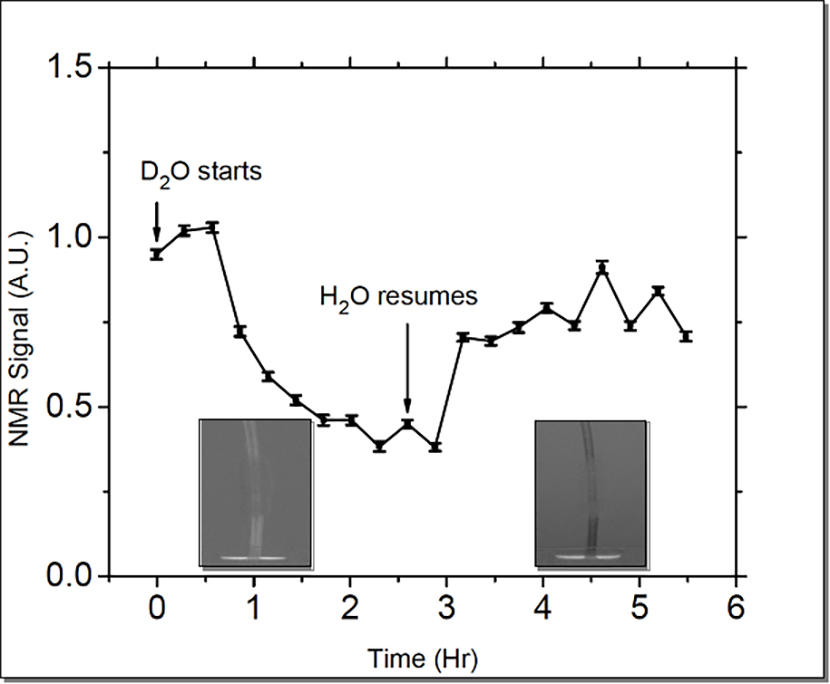
Figure 1. (Left panel): Photograph of the NMR and neutron beam set-up. Neutrons enter at right, the NMR system surrounds the juniper branch, and the neutron detector is at left. (Right panel) Inset shows a neutron radiography image of the set-up. The branch is in the middle, the bars on both sides are the NMR shield, and the thickening on the branch is the NMR coil.
The Los Alamos research team for the combined ULF-NMR and neutron imaging studies included: Sanna Sevanto (EES-14), Michelle Espy and Michael Malone (P-21), L. Turin Dickman (EES-14), James Hunter (AET-6), Ron Nelson (P-27), Jacob Yoder (C-IIAC), and Sven Vogel (MST-8).
The Laboratory Directed Research and Development (LDRD) program funded the work, which supports the Lab’s Global Security mission area and the Science of Signatures science pillar through the capability to monitor the impact of drought on vegetation. A PADSTE (Principal Associate Directorate for Science, Technology, and Engineering) small equipment grant purchased the neutron imaging equipment. NNSA Weapons Campaign 8 (Enhanced Surveillance) sponsored the development and operation of the neutron imaging detector. Technical contact: Sanna Sevanto and Michelle Espy
Ultracold Neutron Source at LANSCE enables new experiments Los Alamos researchers employ capabilities at the Los Alamos Neutron
Los Alamos researchers employ capabilities at the Los Alamos Neutron Science Center (LANSCE) User Facility to design and construct new experimental instruments for studies to produce a better understanding of the fundamental forces at work in the universe. These experiments rely on the power and flexibility of the LANSCE Ultracold Neutron (UCN) Source, which offers a high density of ultracold neutrons with the world’s highest degree of polarization and low background from the spallation source.
Neutron electric dipole moment experiment

Figure 3. New ultracold neutron guide fills in gaps where a major source of ultracold neutrons is lost.
The project is developing a new nEDM experiment with a sensitivity of 3x10-27 e-cm, a 10-fold improvement over the current limit. Performing this experiment requires a stored neutron density of approximately 100 UCN/cc. Achieving this density necessitated an improved neutron guide to transport neutrons from the source to the experimental measurement area. Los Alamos researchers developed, fabricated, and tested a new design to provide the improved performance required for the nEDM experiment.
Making a joint between guide components with a minimum gap has been a challenge in constructing a neutron transport system. Even a gap of a few tenths of a millimeter for every meter of a guide section leads to a significant loss of transported neutrons. The team based the new Los Alamos guide on the standard conflat flange design and modified it to minimize the gap between adjacent guide sections. Scientists stored the ultracold neutrons in guide sections and measured the lifetime of the stored ultracold neutrons (approximately 100 seconds), revealing a significant improvement over similar previous measurements using the current guide joint design.

Figure 4. (Top): The multilayer boron-10 (10B) surface detector for ultracold neutrons consists of a thin-film 10B top layer supported by a luminescent layer of zinc sulfide: silver (ZnS:Ag). One of the charged particles α or lithium-7 (7Li) generated from the neutron capture stops in the ZnS:Ag layer and emits light that passes through a light-guide or a transparent window before detection by photomultipliers (PMT). A 10B thickness of 100 nm and a ZnS:Ag thickness of a few microns are sufficient for this purpose.
Ultracold neutron tau experiment
Systematic errors due to ultracold neutron-material surface interactions have limited the measurement accuracy. Several experiments have given conflicting answers, deviating from each other by more than five sigmas. Los Alamos, Indiana University, and other collaborators have built the world’s first magnetic bottle to store ultracold neutrons. The bottle completely removes the ultracold neutron-surface interactions to provide a new venue to determine the neutron lifetime. Several detector technologies have been developed at Los Alamos for this unique experiment, including a new multilayer surface detector for ultracold neutrons (Figure 4). This detector replaces existing ultracold neutron detectors and has been deployed for ultracold neutron flux monitoring, development of ultracold neutron guides, and ultracold neutron lifetime research.
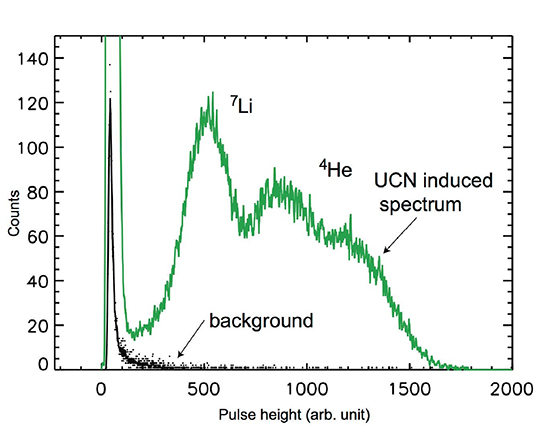
Figure 5. Examples of the pulse height spectra with ultracold neutrons (green trace) and without ultracold neutrons (black trace). The peaks associated with the ultracold spectrum are related to the characteristic energies of α and 7Li from the 10B(n, α)7Li reaction. Helium-4 is 4He. The 7Li peak is fitted with a skewed Gaussian function.
Figure 5 depicts the sample spectra. Researchers collected a background spectrum without ultracold neutrons present (black spectrum) and with the Ultracold Neutron Source (green spectrum). The signal peaks from the 7Li and alpha particles are easily seen. Existing helium-3 (3He) and 10B detectors verified the high ultracold neutron detection efficiency and low background.
With these improvements in place, researchers plan to measure the neutron lifetime with unprecedented accuracy. These capabilities will also enable new experimental studies of physics beyond the standard model.
Lead experimenters are Takeyasu Ito, Mark Makela, John Ramsey, Robert Pattie, Zhaowen Tang, Zhehui Wang, Chris Morris, and Nathan Callahan (Subatomic Physics, P-25); Mark Hoffbauer (Chemical Diagnostics and Engineering, C-CDE); and UCNτ principal investigator Susan Seestrom (Physics, P-DO).
The Laboratory Directed Research and Development (LDRD) program funded both experiments, which support the Laboratory’s Global and Energy Security mission areas and Nuclear and Particle Futures science pillar. Technical contacts: Takeyasu Ito (nEDM) and Zhehui Wang (UCNτ)
Materials Physics and Applications
Accessing optoelectronic properties in atomic layered semiconducting materials
A Los Alamos-led collaboration investigated an emerging class of two-dimensional nanomaterials beyond graphene, called transition-metal dichalcogenides. These materials have the formula have the formula ME2, where M is a transition metal and E is sulfur, selenium, or tellurium. The researchers discovered how to access the intrinsic optoelectronic properties of transition-metal dichalcogenides. The materials could enhance high-performance semiconductor devices including those for energy harvesting applications. The journal ACS Nano published their findings.
Transition-metal dichalcogenides materials were developed to overcome the semi-metallic nature of graphene (an atomic layer-thick material made from carbon) that limits its application in optoelectronics. The transition-metal dichalcogenides exhibit relatively high mobility of carriers and enhanced light-matter interactions when reduced to a monolayer (0.6 nm). These properties are attractive for optoelectronic applications. However, the device performances have been hindered by the native energy barriers at the interfaces between transition-metal dichalcogenides and metal electrodes, which limit the extraction of charge carriers.
One of the chief challenges of transition-metal dichalcogenide research is to achieve devices with ohmic contacts, thereby gaining access to optoelectronic properties that cause devices to function without the extrinsic effects that could limit their performances. A research team from Los Alamos, Rutgers University, Rice University, and Pacific Light Technologies has measured the optoelectronic properties of transition-metal dichalcogenides. After a semiconducting to metallic phase transformation occurred in regions underneath the metal electrodes, the scientists could access the otherwise masked, intrinsic optoelectronic response of transition-metal dichalcogenides.
This research builds on previous Los Alamos-directed work on phase transformation to achieve low resistance contacts to atomic layered transition-metal dichalcogenides. The results, achieved at the Center for Integrated Nanotechnologies (CINT) at Los Alamos, are important for the fundamental understanding of intrinsic optoelectronic properties of atomically thin transition-metal dichalcogenides where ohmic contacts are necessary to achieve high-efficiency devices with low power consumption or minimum energy loss.
In the new research, the team used photoexcited molybdenum disulfide (MoS2). They characterized the material via correlated scanning photocurrent microscopy and photoluminescence imaging. The results demonstrated that a reduction of native Schottky barriers by phase transformation (from 200 meV down to few meV) enhances the photoresponsivity of a device by more than one order of magnitude. This is a crucial parameter in achieving high-performance optoelectronic devices. The study provides insights into the efficient and optimum design of high performance transition-metal dichalcogenide-based devices via simple and reliable procedures for forming ohmic-like contacts to access intrinsic optoelectronic properties.
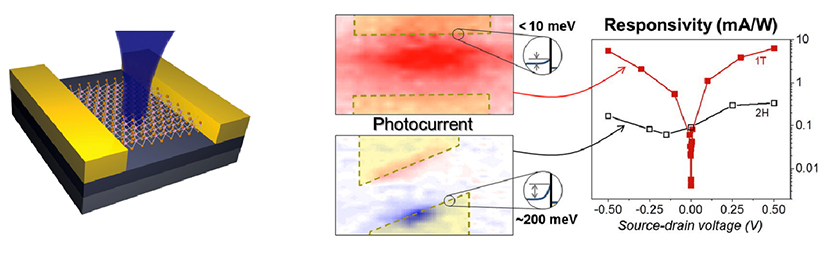
Figure 6. Spatially resolved intrinsic photocurrent response from MoS2. (Left sketch): The measurements were performed using a scanning photocurrent microscopy technique where a focused laser beam rasters on the sample surface and electrical response is measured. While maximum photocurrent generation occurs at the MoS2/metal contacts for a conventional device (bottom middle map), after the metallic phase transformation of regions underneath the metal electrodes, the photocurrent peak is observed towards the center of the device channel (top middle map), suggesting a strong reduction of native Schottky barriers. Circular insets illustrate Schottky barrier height derived from the optoelectronic measurements. The photo-responsivity of MoS2 devices was enhanced by at least one order of magnitude after phase transformation (red) as compared with their conventional counterparts (black) (right graph).
Reference: “Spatially Resolved Photoexcited Charge-Carrier Dynamics in Phase-Engineered Monolayer MoS2,” ACS Nano 9, 840 (2015): doi: 10.1021/nn506469v. Authors include: Hisato Yamaguchi, Gautam Gupta, and Aditya Mohite (Materials Synthesis and Integrated Devices, MPA-11); Jean-Christophe Blancon and Jared Crochet (Physical Chemistry and Applied Spectroscopy, C-PCS); Rajesh Kappera and Manish Chhowalla (Rutgers University); Pulickel Ajayan and Jun Lou (Rice University); and Benjamin D. Mangum (Pacific Light Technologies). Jon Baldwin (MPA-CINT) performed metal electrode depositions, Chris Sheehan (MPA-CINT) conducted electron microscopy, and Darrick William (MPA-CINT) maintained the SEM system for e-beam lithography.
The Laboratory Directed Research and Development (LDRD) program funded the work. The research was performed in part at the Center for Integrated Nanotechnologies (CINT), a DOE Office of Science User Facility that Los Alamos National Laboratory and Sandia National Laboratories operate for the DOE. Yamaguchi received a LANL Director’s Postdoctoral Fellowship.
The effort supports the Lab’s Energy Security mission area and the Materials for the Future science pillar through research to make more energy efficient semiconductor devices, including those for energy harvesting applications. Technical contact: Aditya Mohite
Materials Science and Technology
Scientists examine a surrogate for PuO2 in nuclear fuel studies
For decades, scientists have used cerium dioxide (CeO2) in the laboratory as a surrogate material to explore the synthesis, properties, and performance of nuclear fuel forms, or processes involving plutonium dioxide (PuO2), such as mixed oxide fuel. Unlike plutonium or uranium, CeO2 is not radioactive; therefore, it is easier and less costly to use it in laboratories. Yet until the Japan Atomic Energy Agency (JAEA) and Los Alamos researchers conducted experiments at the Los Alamos Fuels Research Laboratory (FRL), no one had investigated how accurate or inaccurate CeO2 is as a surrogate. The team learned that the most significant differences are in the thermochemistry between a (U,Ce)O2 surrogate and a (U,Pu)O2 fuel. The Journal of the American Ceramic Society published their findings.
Cerium dioxide reduces much more readily than PuO2 does. Even the oxygen content of ambient air is insufficient to prevent oxygen vacancies from building into the lattice over time at room temperature. Although this difference has been qualitatively understood, the extent to which these vacancies degraded the properties of CeO2 as a function of temperature was unknown previously. The thermal conductivity of oxide materials is particularly sensitive to structural defects, and its importance to the design of nuclear fuels and reactor operation is critical.
The Los Alamos-Japan team conducted experiments at the Fuels Research Laboratory to determine the thermal conductivity of stoichiometric CeO2 based on high-temperature measurements of thermal expansion, thermal diffusivity, and heat capacity. They calculated the product of density, thermal diffusivity, and heat capacity to determine the thermal conductivity of stoichiometric CeO2. The scientists measured each these three parameters as a function of temperature by dilatometry, laser flash analysis, and differential scanning calorimetry, respectively.
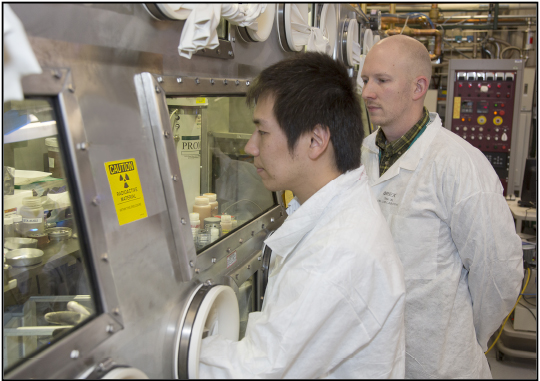
Photo. Shun Hirooka (Japan Atomic Energy Agency) and Andrew Nelson (Polymers and Coatings, MST-7) conduct experiments in a glove box in the Los Alamos Fuels Research Laboratory. Three scientists from the Japan Atomic Energy Agency have come to the Los Alamos Fuels Research Laboratory, which offers the capabilities needed for experiments aimed at understanding what happens to nuclear fuels during atypical conditions, such as the 2011 meltdown at the Fukushima nuclear complex.
The research team compared their results with the existing literature data for CeO2, PuO2, and uranium dioxide (UO2), the most widely used nuclear fuel today. How important the differences are depends on the goal of an investigator’s research. The team identified the following important differences: 1) the thermophysical properties of CeO2 did not match those of PuO2, and 2) the thermal conductivity of CeO2 is generally comparable to UO2 below 1673 K, but not at higher temperatures. This finding might make CeO2 an acceptable surrogate UO2-based composite material where off-stoichiometry could be controlled.
This paper is the first publication resulting from an ongoing collaboration between Los Alamos National Laboratory and the Japan Atomic Energy Agency. In the wake of the accident at the Fukushima Daiichi Nuclear Power Station, which used uranium dioxide fuel, the Japan Atomic Energy Agency has sent one scientist a year to LANL’s Fuels Research Laboratory as part of a bilateral technical collaboration tasked with improving the safety of nuclear energy and providing science-based guidance for cleanup activities at Fukushima.
The Civil Nuclear Energy R&D Working Group was formed between the United States and Japan to conduct cooperative nuclear energy research and development in such areas as advanced reactor and fuel cycle technologies and existing reactor fleet sustainability. Under that umbrella organization, the Fuel Cycle R&D and Waste Management sub-working group project was created in 2013. The nuclear fuels work is performed at Los Alamos through that project.

Figure 7. Thermal conductivity data for CeO2 determined at Los Alamos compared with the accepted fit for UO2 and available data for PuO2. The theoretical densities for each set of data are shown in the legend.
The Japan Atomic Energy Agency and Los Alamos collaborators are studying the U-Ce-O system and the thermophysical properties of its constituents. They are performing broad studies to understand what oxygen activity is required to drive a specific off-stoichiometry in (U,Ce)O2 surrogates. The team measures properties such as thermal conductivity as a function of cerium content and off-stoichiometry. As the collaborators better understand both the thermochemistry and thermophysical properties of (U,Ce)O2, they could execute expansive studies at the Los Alamos Fuels Research Laboratory to obtain detailed insight into how this system works. Once they have experimental data and appropriate models for (U,Ce)O2, they could identify three to four key
Reference: “An Evaluation of the Thermophysical Properties of Stoichiometric CeO2 in Comparison to UO2 and PuO2,” Journal of the American Ceramic Society 97, (11) 3652 (2014); doi: 10.1111/jace.13170. Authors include: Andy Nelson, Dylan Rittman, Joshua White, and John Dunwoody (Polymers and Coatings, MST-7); Masato Kato (Japan Atomic Energy Agency); and Kenneth McClellan (Materials Science in Radiation and Dynamics Extremes, MST-8).
To read more about the U.S.-Japan bilateral collaboration:
The DOE Office of Nuclear Energy Fuel Cycle Research and Development program funded the LANL work, and the DOE Nuclear Energy University Program supported portions of the research. The work benefited from the use of the Los Alamos Fuels Research Laboratory, which the DOE Office of Nuclear Energy and the National Nuclear Security Administration sponsor. The research supports the Laboratory’s Energy Security and Global Security mission areas and Materials for the Future science pillar through the ability to conduct research on nuclear fuels. Technical contact: Andy Nelson
Mesoscale Materials Science on the Roadmap to MaRIE
Modeling quasi-brittle damage behavior in metals
Los Alamos National Laboratory scientists have developed a new material strength and damage model that accounts for brittle-ductile transitions in the mechanical response of quasi-brittle metals (materials that show measurable deformation prior to failure). The model accounts for fracture behavior by evolving tensile and compressive damage separately using energy-based criteria and tensor representation theory. This continuum-scale material model is unique because it accounts for mesoscale deformation and damage mechanisms. For example, strength and plasticity are built on a processed-based, viscoplastic framework that can account for mechanisms such as deformation twinning.
The Lab researchers have implemented this novel model into Lagrangian Applications Project (LAP) codes. This framework enables the study of brittle failure and brittle-ductile transitions in the material response, something that has not been previously possible in macro-scale continuum codes at LANL. Because tensile and compressive damage are evolved separately, visualization of mesoscale deformation mechanisms such as crack formation and shear banding is possible (Figure 8). Due to the viscoplastic framework, strength and plasticity responses in this model produce smooth transitions between the elastic and plastic regimes of stress-strain curves. Early comparisons of simulation results with flyer-plate experimental data are favorable.
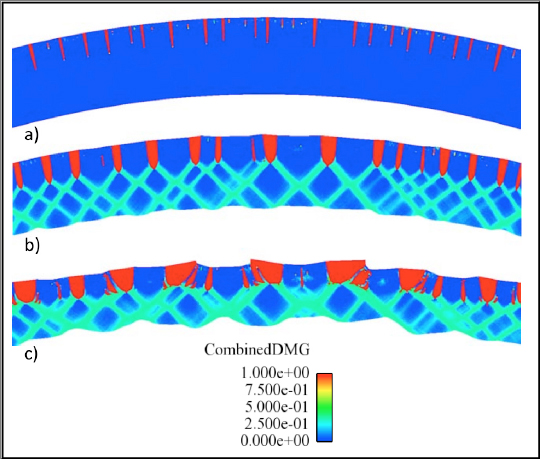
Figure 8. Damage evolution of a section of a thin walled beryllium cylinder with a constant internal pressure applied at times: a) 10.0 s, b) 18.5 s, c) 25.5 s. The red regions show tensile damage, specifically crack formation. Green regions show shear band formation.c
This work is an example of Science on the Roadmap to MaRIE, the Laboratory’s proposed experimental facility for Matter-Radiation Interactions in Extremes. This model provides an example of a computational tool that could be informed and validated by MaRIE’s experimental results. Such a connection between modeling and experimental efforts would enhance LANL’s understanding of material behavior under extreme conditions and provide a distinctive capability to predict overall material response. More information: http://www.lanl.gov/science-innovation/science-facilities/marie/_assets/docs/roadmap-to-marie1.pdf
Researchers include: Abigail Hunter (Computational Physics, XCP-1), Alek Zubelewicz (retired from Physics and Chemistry of Materials, T-1), and Esteban Rougier (Geophysics, EES-17).
The NNSA Advanced Simulation and Computing (ASC) Program funded the work through the Integrated Codes (IC) and Physics and Engineering Models (PEM) program elements. This research supports the Lab’s Nuclear Deterrence mission area and the Materials for the Future and Information, Science, and Technology science pillar by providing a predictive tool that accounts for the effects of brittle-ductile transitions in material response. Technical Contact: Abigail Hunter